Polarized spectroscopic properties of Ho3+ ions in NaLa(MoO4)2 crystal
Optical Materials(2011)
摘要
A Ho 3+ -doped NaLa(MoO 4 ) 2 single crystal was grown by the Czochralski method. The polarized absorption spectra, polarized fluorescence spectra, and fluorescence decay curves of the crystal were measured at room temperature. The spontaneous emission probabilities, radiative lifetimes, and fluorescence branching ratios of the typical fluorescence multiplets of Ho 3+ ions were calculated. The polarized stimulated emission and gain cross-sections of the 5 I 7 → 5 I 8 transition were obtained. The results show that the Ho 3+ :NaLa(MoO 4 ) 2 crystal is a promising gain medium for tunable and ultrashort pulse lasers operating around 2.0 μm. Keywords Ho 3+ :NaLa(MoO 4 ) 2 crystal Polarized spectroscopic properties 1 Introduction Ho 3+ ion in solids has an energy level scheme with various gaps and then rich emission spectral lines in ultraviolet, visible, and infrared regions. Therefore, various Ho 3+ -doped materials have been investigated as gain media for up-conversion visible laser [1,2] and infrared laser around 2.0 and 2.9 μm [3,4] . The visible laser has many attractive applications, such as color display, data storage, and undersea communication. The infrared laser has potential applications in medicine, optical communication, and lidar. The NaLa(MoO 4 ) 2 (NLM) crystal belongs to the compounds with a general formula MT ( X O 4 ) 2 ( M = Na + or Li + ions, T = Gd 3+ , Y 3+ , La 3+ or Bi 3+ ions, and X = W 6+ or Mo 6+ ions), which have been proved to have a locally disordered tetragonal crystallographic structure with space group I 4 ¯ , in which T 3+ can randomly occupy two different sites, 2 b and 2 d [5–7] . The disordered structure of this kind of crystals will induce the inhomogeneous broadening of optical spectra bands when optically active ions are doped in this crystal and occupy the positions of T 3+ cations. Therefore, the broadening spectral bands are in favor of tunable and ultrashort pulse laser output. The laser operations of Yb 3+ , Nd 3+ and Tm 3+ -doped NLM crystals have been achieved [8–10] . Especially, multiwave lasers operating simultaneously at wavelengths of 1065, 1176, and 1313 nm with nanosecond pulse duration have also been realized in Nd 3+ :NLM crystal by means of self-SRS [11] . To the best of our knowledge, only some primary spectral parameters of Ho 3+ ions in the NLM single crystal have been presented based on unpolarized spectra [12,13] . In this paper, the polarized spectroscopic properties of the Ho 3+ :NLM crystal are reported. On the basis of the Judd–Ofelt (J–O) theory [14,15] , the spontaneous emission probabilities, radiative lifetimes, and fluorescence branching ratios of Ho 3+ ions in the NLM crystal are calculated. Finally, the 2.0 μm laser properties of the Ho 3+ :NLM crystal are evaluated. 2 Experimental A Ho 3+ :NLM single crystal was grown by the Czochralski method. The stoichiometric amounts of raw materials were accurately weighed according to the formula of NaLa 0.98 Ho 0.02 (MoO 4 ) 2 . The growth procedure was similar to that reported in Ref. [16] . A Ho 3+ :NLM crystal with high optical quality and dimensions up to ∅22 mm × 25 mm was obtained and is shown in Fig. 1 . The concentration of Ho 3+ ions in this crystal was measured to be 0.55 × 10 20 cm −3 (0.92 at.%) by the inductively coupled plasma atomic emission spectrometry (ICP-AES, Ultima2, Jobin–Yvon). Taking the 2.0 at.% Ho 3+ concentration in the raw material into account, the segregation coefficient of Ho 3+ ions in NLM crystal is 0.46. A cuboid sample with dimensions of 6.0 × 5.5 × 4.0 mm 3 ( c × a × a ) was cut from the grown crystal and then polished for spectral experiments. All the spectral experiments were carried out at room temperature. The polarized absorption spectra in the range of 370–2300 nm were recorded using a spectrophotometer (Lambda 900, Perkin–Elmer). The polarized fluorescence spectra in the range of 460–1400 nm and the fluorescence decay curve at 541 nm, corresponding to the 5 S 2 + 5 F 4 → 5 I 8 transition of Ho 3+ ions, were recorded by a spectrometer (FLS920, Edinburgh) when the sample was excited at 452 nm. A xenon lamp and a nanosecond hydrogen-filled flash lamp (nF900, Edinburgh) were used as the excitation sources respectively. The fluorescence signals were detected by a photomultiplier tube (PMT) (R955, Hamamatsu) in the visible region and another PMT (R5509, Hamamatsu) in the near infrared (NIR) region. The polarized fluorescence spectra in the range of 1870–2130 nm and the fluorescence decay curve at 2000 nm, corresponding to the 5 I 7 → 5 I 8 transition of Ho 3+ ions, were recorded by another spectrometer (FSP920C, Edinburgh) with an InSb detector when the sample was excited at 1156 nm. A tunable mid-band OPO laser (Vibrant 355II, Opotek) was used as the excitation source. 3 Results and discussion 3.1 Absorption spectra The room-temperature polarized absorption spectra for the Ho 3+ :NLM crystal are shown in Fig. 2 , where σ and π polarizations are defined in terms of the electric field direction of the incident light being perpendicular and parallel to the c -axis of the Ho 3+ :NLM crystal, respectively. The polarized absorption cross-section could be obtained by: (1) σ GSA q ( λ ) = 2.303 × OD q ( λ ) LN 0 , where q indicates the polarization of the absorption spectra, OD q ( λ ) is the absorbance for q polarization at wavelength λ , L is the thickness of the crystal, and N 0 is the Ho 3+ concentration in the crystal. There are nine absorption bands in each polarized spectrum and all of them can be assigned to the 4 f –4 f transitions of Ho 3+ ions. Because the absorption edge of the NLM crystal is about 400 nm, the bands for the transitions from the ground state 5 I 8 to the exited multiplets above 3 G 5 are covered by the absorption of the host crystal. The absorption cross-sections for π polarization are generally larger than those for σ polarization. The transition of 5 I 8 → 5 I 4 is too weak to be observed. The J–O theory is a popular method for estimating the spectroscopic parameters of rare-earth ions in crystals and glasses [14,15] . The J–O intensity parameters Ω t ( t = 2, 4, 6) can be calculated from the measured absorption spectra. For the 5 I 8 → 5 I 7 transition of Ho 3+ ions, the probabilities of the magnetic-dipole (MD) and electric-dipole (ED) transition are comparable [17] . Therefore, the MD transition line strength has been subtracted from the absorption band of 5 I 8 → 5 I 7 before the J–O calculation [18] . The detailed calculating procedure is similar to that reported in Ref. [19] . The reduced matrix elements of unit tensor operators used in the calculations can be found in Ref. [18] . The refractive index could be calculated by the approximate Sellmeier equation [20] : (2) n 2 ( λ ) = 1 + S λ 2 λ 2 - λ 0 2 where n is the refractive index at wavelength λ , S and λ 0 2 are the Sellmeier coefficients, and λ is in the unit of micrometer. In Ref. [20] constants A = 1/ S and B = λ 0 2 / S have been determined by fitting the measured refractive indices at different wavelengths for ordinary and extraordinary lights, i.e. σ and π polarizations respectively, with Eq. (2) . However, the values of B in Ref. [20] may be wrongly written due to the error of publishing. After re-fitting the experimental data and comparing with the unpolarized Sellmeier equation proposed in Ref. [13] , the reasonable Sellmeier equations were obtained and used for calculating the refractive indices in our work, (3) n σ 2 = 1 + 2.7778 λ 2 / ( λ 2 - 0.0274 ) , (4) n π 2 = 1 + 2.7778 λ 2 / ( λ 2 - 0.0266 ) . The experimental and calculated oscillator strengths f exp and f cal , respectively, and the mean wavelengths λ ¯ of the various absorption bands for σ and π polarizations are listed in Table 1 . The root-mean-square deviations (RMS Δ f ) between f exp and f cal and the relative errors are also listed in this table. From the calculated Ω t parameters of each polarization for the anisotropic crystal, the effective intensity parameters could be calculated by [21] : (5) Ω t ,eff = ( 2 Ω t , σ + Ω t , π ) / 3 . The values of the parameters are listed and compared with those of other Ho 3+ -doped crystals in Table 2 . The Ω 2,eff of the Ho 3+ :NLM crystal is far larger than that reported by Stevens et al. [13] . However, it can also be found from Table 2 that the Ω 2 , eff in this work is similar to those of Ho 3+ -doped tetragonal double molybdate and tungstate crystals such as NaBi(MoO 4 ) 2 [25] , LiBi(MoO 4 ) 2 [25] , NaY(MoO 4 ) 2 [26] , and NaLa(WO 4 ) 2 [27] . Therefore, the result in this work may be more reasonable. Subsequently, the spontaneous emission probabilities of electric-dipole transition A ed q , the magnetic-dipole transition A md , the total spontaneous emission probabilities A = 2 A ed σ + A md + A ed π + A md / 3 , the fluorescence branching ratios β , and the radiative lifetimes τ r of some principal fluorescence multiplets for the Ho 3+ :NLM crystal can be estimated and the values are listed in Table 3 . The detailed calculating procedure is similar to that reported in Ref. [19] . 3.2 Fluorescence spectra Fig. 3 shows the room-temperature polarized fluorescence spectra in the range of 460–1400 nm under excitation at 452 nm, i.e., the 5 G 6 + 5 F 1 multiplets is populated. It can be seen that fluorescence for π polarization is generally more intensive than that for σ polarization, which is in agreement with the calculated polarized radiative transition probabilities listed in Table 3 . Each spectrum consists of four bands in the visible region around 489, 539, 657, and 753 nm, which can be assigned to the transitions of 5 F 3 → 5 I 8 , 5 S 2 + 5 F 4 → 5 I 8 , 5 F 5 → 5 I 8 , and 5 S 2 + 5 F 4 → 5 I 7 , respectively. Three weak bands were also detected in the NIR region around 983, 1014, and 1189 nm, which can be assigned to the transitions of 5 F 5 → 5 I 7 , 5 S 2 + 5 F 4 → 5 I 6 , and 5 I 6 → 5 I 8 , respectively. The energy gaps between 5 G 6 + 5 F 1 and 5 F 2 + 3 K 8 , 5 F 2 + 3 K 8 and 5 F 3 , and 5 F 3 and 5 S 2 + 5 F 4 are about 900, 800, and 2100 cm −1 , respectively, and the maximum phonon energy of the (MoO 4 ) 2− group is about 890 cm −1 [28] . Therefore, after the 5 G 6 + 5 F 1 multiplets was excited, only very weak fluorescence from the 5 F 3 multiplet was observed and the 5 S 2 + 5 F 4 multiplets were populated immediately by the multi-phonon relaxation. Under excitation at 1156 nm, the Ho 3+ ions were excited to the 5 I 6 multiplet and the room-temperature polarized fluorescence spectra of the Ho 3+ :NLM crystal were recorded in the range of 1870–2130 nm. The polarized emission cross-section of the 5 I 7 → 5 I 8 transition can be calculated by the Fuchtbauer–Ladenburg (F–L) formula [29] from the polarized fluorescence spectra: (6) σ EM q ( λ ) = A q ( J → J ′ ) λ 5 I q ( λ ) 8 π cn 2 ∫ λ I q ( λ ) d λ , where I q ( λ ) is the fluorescence intensity at wavelength λ for q polarization, c is the velocity of light. The wavelength dependences of the polarized absorption cross-section of the 5 I 8 → 5 I 7 transition (see Fig. 2 ) and the polarized emission cross-section of the 5 I 7 → 5 I 8 transition derived by the F–L formula are shown in Fig. 4 . The peak emission cross-sections of the 5 I 7 → 5 I 8 transition are about 0.89 × 10 −20 and 1.43 × 10 −20 cm 2 for σ and π polarizations, respectively, and both at 2040 nm. The full widths at half maximum (FWHMs) of the emission bands for σ and π polarizations are 60 and 50 nm, respectively. The peak emission cross-section of the Ho 3+ :NLM crystal for π polarization are slightly smaller than those of the Ho 3+ :YAG (1.59 × 10 −20 cm 2 at 2090 nm) [30] and Ho 3+ :YLF (1.55 × 10 −20 cm 2 for π polarization at 2050 nm) [31] crystals, in which the 2.0 μm laser has been realized [32,33] . The tunable range of the laser via the 5 I 7 → 5 I 8 transition can be evaluated from the polarized gain curve defined as: (7) σ GAIN q ( λ ) = P σ EM q ( λ ) - ( 1 - P ) σ GSA q ( λ ) , where P represents the proportion of population inversion. Fig. 5 shows the polarized gain curves for several values of P in the 1970–2130 nm spectral region. The curves at wavelength longer than 2080 nm are obscure due to the low signal-to-noise ratio of the absorption spectra. For P = 0.5, the peak σ GAIN q values are about 0.22 × 10 −20 and 0.33 × 10 −20 cm 2 for σ and π polarizations, respectively, and both at 2044 nm. The positive gains for P = 0.5 are in a range from 1984 nm to 2128 nm for σ polarization and from 1976 nm to 2129 nm for π polarization. The results suggest that the Ho 3+ :NLM crystal is a potential tunable and ultrashort pulse laser material operating near 2.0 μm. 3.3 Fluorescence dynamics Because the fluorescence bands for the transitions from the 5 S 2 and 5 F 4 multiplets to 5 I 8 multiplet overlapped, as shown in Fig. 3 , the fluorescence decay signal of these two multiplets 5 S 2 + 5 F 4 was detected together. The decay curve at 541 nm, corresponding to the transitions from those two multiplets is shown in Fig. 6 in semi-log scale. By linear fitting, the fluorescence lifetime τ f of the 5 S 2 + 5 F 4 multiplets was derived to be 4.0 μs, which is much shorter than the radiative lifetimes τ r of the 5 S 2 and 5 F 4 multiplets listed in Table 3 . This is mainly caused by the rapid multi-phonon relaxation from the 5 S 2 multiplet to the next lower 5 F 5 multiplet, because the energy gap between them is only ∼2800 cm −1 . Because 5 I 8 is the ground multiplet, the emission of the 5 I 7 → 5 I 8 transition can be reabsorbed. In order to reduce the effect of radiation trapping on the measurement of fluorescence lifetime of the 5 I 7 multiplet, the decay curve for a powder sample was also measured. A piece of the Ho 3+ :NLM crystal was ground into a powder sample and then immersed into monochlorobenzene fluid with refractive index 1.52 contained in a glass cuvette, which was used as refractive index matching fluid to reduce the radiation trapping effect [34] . The decay curves measured from the powder and bulk samples are shown in Fig. 7 in semi-log scale and both of them display a single-exponential behavior. By linear fitting, the fluorescence lifetime of the powder sample is 5.47 ms and shorter than the 7.20 ms of the bulk sample, which reveals that the fluorescence lifetime of the 5 I 7 multiplet is affected by the radiation trapping effect. In fact, the above phenomenon has also been observed in other Ho 3+ -doped crystals such as Ho 3+ :PbWO 4 [35] and Ho 3+ :Y 2 O 3 [36] . From the fluorescence lifetime τ f measured from the powder sample and the radiative lifetime τ r of the 5 I 7 multiplet listed in Table 3 , the fluorescence quantum efficiency of this multiplet, η = τ f τ r , was estimated to be 83%. The high quantum efficiency reveals that the non-radiative transition from the 5 I 7 multiplet of the 0.92 at.% Ho 3+ :NLM crystal is weak. This is mainly caused by the low phonon energy of the (MoO 4 ) 2− group (about 890 cm −1 ) [28] compared with the large energy gap (about 5200 cm −1 ) between the 5 I 7 and 5 I 8 multiplets. Thereby, the thermal load caused by the non-radiative transition from the upper laser level 5 I 7 in Ho 3+ :NLM crystal can be reduced dramatically during the 2.0 μm laser operation. In addition, the threshold for laser oscillation can also be reduced [37] . Both of the results indicate that Ho 3+ :NLM crystal is a promising candidate for gain medium of 2.0 μm laser. 4 Conclusions A Ho 3+ -doped NLM crystal was grown by the Czochralski method. The polarized absorption spectra, polarized fluorescence spectra, and fluorescence decay curves of the grown crystal were measured at room temperature. The effective intensity parameters calculated from the room-temperature absorption spectra on the basis of J–O theory are Ω 2 , eff = 16.19 × 10 −20 cm 2 , Ω 4 , eff = 4.21 × 10 −20 cm 2 , and Ω 6 , eff = 1.00 × 10 −20 cm 2 . The polarized emission and gain cross-sections of the 5 I 7 → 5 I 8 transition were calculated to evaluate the properties of the 2.0 μm laser operation. The broad emission band around 2.0 μm indicates that the Ho 3+ :NLM crystal can be used to realize tunable and ultrashort pulse laser output via the 5 I 7 → 5 I 8 transion. The fluorescence lifetimes of the 5 S 2 + 5 F 4 and 5 I 7 multiplets are about 4.0 μs and 5.47 ms, respectively. The quantum efficiency of the 5 I 7 multiplet is about 83%. The spectral parameters related to 2.0 μm laser of the Ho 3+ :NLM crystal are similar to those of the Ho 3+ :YAG and Ho 3+ :YLF crystals, in which efficient 2.0 μm laser operations have been realized. All of the above results indicate that the Ho 3+ :NLM crystal is a promising solid-state laser material for 2.0 μm laser with large tunable range. Acknowledgements This work has been supported by the National Natural Science Foundation of China (Grant 50972142), the Major Programs of the Chinese Academy of Sciences (Grant SZD08001-1), and the Chinese National Engineering Research Center for Optoelectronic Crystalline Materials. References [1] L.F. Johnson H.J. Guggenheim Appl. Phys. Lett. 19 1971 44 [2] D.S. Funk S.B. Stevens S.S. Wu J.G. Eden IEEE J. Quantum Electron. 32 1996 7 [3] S. So J.I. Mackenzie D.P. Shepherd W.A. Clarkson J.G. Betterton E.K. Gorton J.A.C. Terry Opt. Express 14 2006 10481 [4] B.M. Walsh Laser Phys. 19 2009 855 [5] C. Cascales M.D. Serrano F. Esteban-Betegón C. Zaldo R. Peters K. Petermann G. Huber L. Ackermann D. Rytz C. Dupré M. Rico J. Liu U. Griebner V. Petrov Phys. Rev. B 74 2006 174114 [6] M. Rico U. Griebner V. Petrov P. Ortega X. Han C. Cascales C. Zaldo J. Opt. Soc. Am. B 23 2006 1083 [7] M. Rico A. Méndez-Blas V. Volkov M.Á. Monge C. Cascales C. Zaldo A. Kling M.T. Fernández-Díaz J. Opt. Soc. Am. B. 23 2006 2066 [8] A.V. Mandrik A.E. Troshin V.E. Kisel A.S. Yasukevich G.N. Klavsut N.V. Kuleshov A.A. Pavlyuk Appl. Phys. B 81 2005 1119 [9] E.V. Zharikov D.A. Lis A.M. Onishchenko V.A. Romanyuk K.A. Subbotin S.N. Ushakov A.V. Shestakov Quantum Electron. 36 2006 39 [10] W.J. Guo Y.J. Chen Y.F. Lin Z.D. Luo X.H. Gong Y.D. Huang J. Appl. Phys. 103 2008 093106 [11] A.V. Gulin V.A. Pashkov N.S. Ustimenko Proc. SPIE 4350 2001 36 [12] A.M. Korovkin A.M. Morozov A.M. Tkachuk A.A. kaminskii Z.L. Morgenshtern D.T. Sviridov Spectroscopy of Crystals 1975 Nauka Moscow 281 [13] S.B. Stevens C.A. Morrison T.H. Allik A.L. Rheingold B.S. Haggerty Phys. Rev. B 43 1991 7386 [14] B.R. Judd Phys. Rev. 127 1962 750 [15] G.S. Ofelt J. Chem. Phys. 37 1962 511 [16] W.J. Guo Y.F. Lin X.H. Gong Y.J. Chen Z.D. Luo Y.D. Huang J. Appl. Phys. 104 2008 053105 [17] W.T. Carnall P.R. Fields K. Rajnak J. Chem. Phys. 49 1968 4412 [18] B.M. Walsh N.P. Barnes B.D. Bartolo J. Appl. Phys. 83 1998 2772 [19] J.H. Huang X.H. Gong Y.J. Chen Y.F. Lin J.S. Liao X.Y. Chen Z.D. Luo Y.D. Huang App. Phys. B 89 2007 73 [20] S.N. Ushakov V.A. Romanyuk P.A. Ryabochkina I.A. Shestakova D.A. Lis K.A. Subbotin A.V. Shestakov E.V. Zharikov Quantum Electron. 40 2010 475 [21] Z.D. Luo X.Y. Chen T.J. Zhao Opt. Commun. 134 1997 415 [22] A.A. Kaminskii Crystalline Lasers: Physical Processes and Operating Schemes 1996 CRC Press Boca Raton [23] S. GoŁab P. Solarz G. Dominiak-Dzik T. Łukasiewicz M. Świrkowicz W. Ryba-Romanowski Appl. Phys. B 74 2002 237 [24] M.C. Pujol J. Massons M. Aguiló F. Díaz M. Rico C. Zaldo IEEE J. Quantum Electron. 38 2002 93 [25] A. Méndez-Blas M. Rico V. Volkov C. Zaldo C. Cascales Phys. Rev. B 75 2007 174208 [26] X.A. Lu Z.Y. You J.F. Li Z.J. Zhu G.H. Jia B.C. Wu C.Y. Tu J. Phys. D: Appl. Phys. 39 2006 3755 [27] Y.P. Wei H.Y. Wang F.G. Yang Z.Y. You J.F. Li Z.J. Zhu Y. Wang C.Y. Tu J. Phys. D: Appl. Phys. 40 2007 5883 [28] V. Ramakrishnan G.A. Dhas P.S. Narayanan J. Raman Spectros. 17 1986 273 [29] B.F. Aull H.P. Jenssen IEEE J. Quantum Electron. 18 1982 925 [30] T.Y. Fan G. Huber R.L. Byer P. Mitzscherlich IEEE J. Quantum Electron. 24 1988 924 [31] B.M. Walsh N.P. Barnes M. Petros J. Yu U.N. Singh J. Appl. Phys. 95 2004 3255 [32] M. Schellhorn A. Hirth C. Kieleck Opt. Lett. 28 2003 1933 [33] E.P. Chicklis C.S. Naiman R.C. Folweiler D.R. Gabbe H.P. Jenssen A. Linz Appl. Phys. Lett. 19 1971 119 [34] S. Guy Phys. Rev. B 73 2006 144101 [35] I.S. Mirov V.V. Fedorov I.S. Moskalev D.V. Martyshkin S.Y. Beloglovski S.F. Burachas Y.A. Saveliev A.M. Tseitline Opt. Mater. 31 2008 94 [36] F. Auzel G. Baldacchini L. Laversenne G. Boulon Opt. Mater. 24 2003 103 [37] Z.D. Luo Y.D. Huang X.Y. Chen Spectroscopy of Solid-State Laser and Luminescent Materials 2007 Nova Science Publishers New York
更多查看译文
关键词
Ho3+:NaLa(MoO4)2 crystal,Polarized spectroscopic properties
AI 理解论文
溯源树
样例
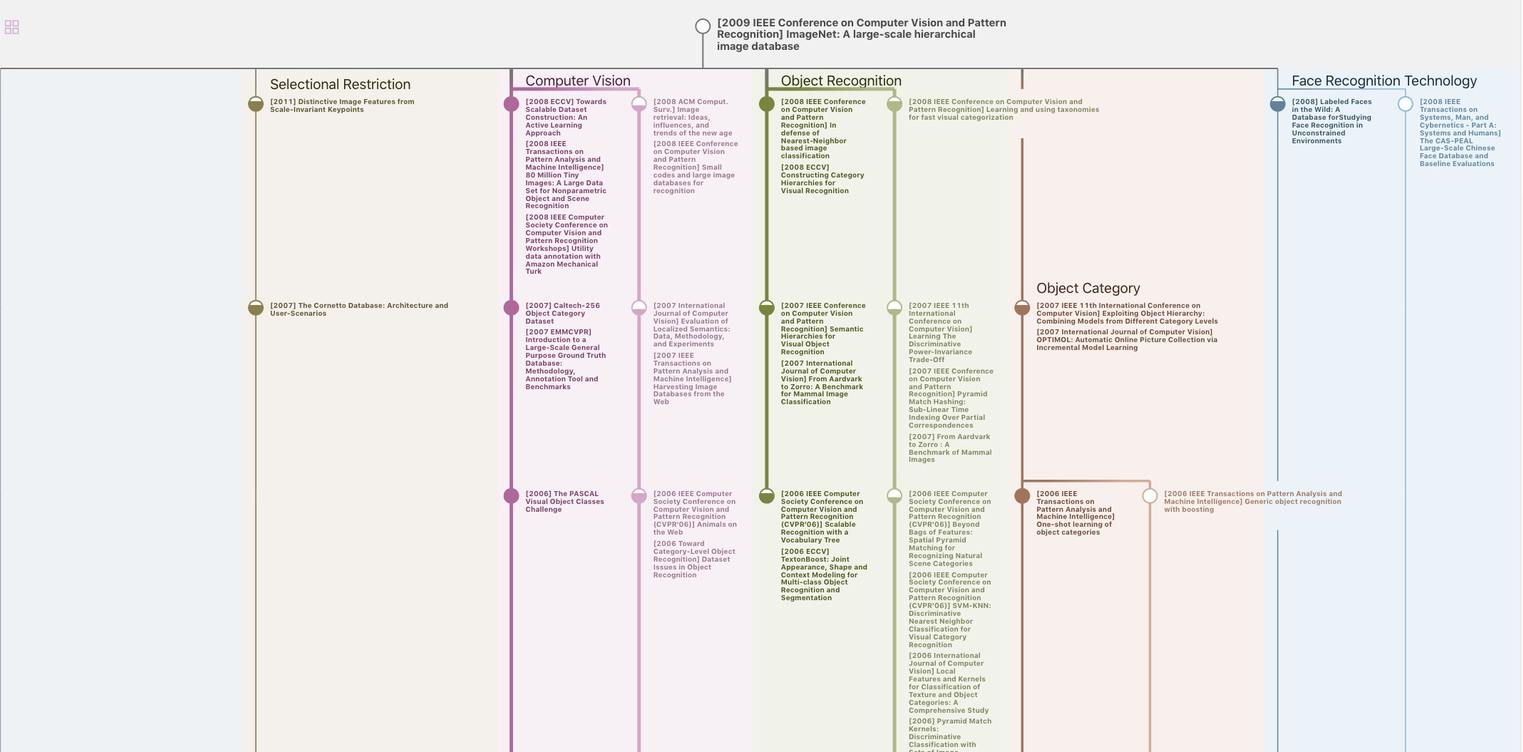
生成溯源树,研究论文发展脉络
Chat Paper
正在生成论文摘要