Lyophilization and enhanced stability of fluorescent protein nanoparticles
Biochemical and Biophysical Research Communications(2011)
摘要
Protein nanoparticles (PNPs) that are nanostructured biomaterials with intrinsic biological function have been widely employed as three-dimensional nanobiomaterials for sensitive bioassays, MRI contrast, semiconductor devices, template for hybrid materials, etc., and stable and long-term maintenance of PNPs seems to be of crucial importance. We evaluated the stability of PNPs and the efficacy of lyophilization for the long-term stability of PNPs, especially using green fluorescent protein nanoparticles (gFPNPs) as a model PNP. Fluorescence intensities and TEM images of gFPNPs were analyzed to monitor their functional and structural stabilities. Unlike the green fluorescent protein monomers (eGFP) that were gradually inactivated in aqueous solution, gFPNP in the same aqueous solution retained the initial fluorescence activity and spherical nanoparticle structure even for 2 weeks at 4 °C. To ensure stable and long-term maintenance of gFPNPs, gFPNPs in aqueous solution were converted to the dried solid forms through lyophilization. It is notable that fluorescence activity and nanoparticle structure of gFPNPs that were lyophilized with both Tween 80 and sucrose were very stably maintained even for 10 weeks at various storage temperatures (−20 °C, 4 °C, 25 °C, and 37 °C). During the period of 10 weeks, the fluorescence of gFPNP was always more than 80% level of initial fluorescence at a wide range of temperature. Although this stability study was focused on gFPNPs, the developed optimal lyophilization conditions for gFPNPs can be applied in general to stable and long-term maintenance of many other PNP-derived biomaterials. Keywords Fluorescent protein nanoparticles Lyophilization Stability Protein nanoparticle structure 1 Introduction Protein nanoparticles (PNPs) with a nano-scale (5–50 nm in diameter) spherical structure that is formed via self-assembly of protein monomers have been used in many applications including diagnostic three-dimensional probes [1–3] , MRI contrast reagents [4,5] , semiconductor devices [6–8] , template for hybrid materials [9–13] , etc. They have unique characteristics such as small size, high surface-to-volume ratio, size uniformity, stable structure, and non-toxicity. We previously applied the engineered PNPs successfully to the highly sensitive diagnostic system [1–3] and the construction of nano-sized architecture [14] . Although the superiority of PNPs has been proven through several applications, however, it has been generally considered that protein-based materials tend to be easily degraded and deformed by heat, extreme pH, and proteases because of their intrinsic labile structure, resulting poor storage stability. In this regard, detailed stability properties and appropriate method for long-term maintenance of PNPs need to be established to enlarge the applicability of PNPs. Lyophilization has been commonly used to formulate the proteins into solid forms to overcome the instability and expand the shelf life of protein-based materials [15] . However freezing and drying processes often cause a denaturation and inactivation of proteins because of high solute concentration, formation of ice crystal, dramatic pH changes, and so on [15] . Thus, stabilizers are often used to protect the proteins from lyophilization-derived stresses. Although lyophilization of proteins was successfully implemented, however, maintenance of stability and activity of lyophilized proteins for a long period still remains as a challenge. To establish the optimal condition for long-term maintenance, hence, stabilizer composition and storage temperature should be carefully considered depending on the target proteins. The 24 subunits of human ferritin heavy chain (hFTN-H) are self-assembled into hollow shell structure (12 nm in diameter) through 4-3-2 symmetry pattern [3,16] . We previously reported that the fusion of enhanced green fluorescent protein (eGFP) to C-terminus of hFTN-H also resulted in the formation of green fluorescent PNPs (gFPNPs) and that gFPNPs maintained its fluorescence even at high temperature (70 °C), in a wide range of pH and in the presence of protein denaturing reagent (GdnHCl) [16] , demonstrating high stability of gFPNPs. This result is also consistent with our recent finding that the fusion mutant of hFTN-H and eGFP that are linked by a flexible glycine-rich sequence showed more enhanced stability in aqueous solution at 25 °C even for 2 weeks [17] . In this study, more systematic and detailed investigations about the stability characteristics and the conditions for maintaining high stability of PNPs were carried out using gFPNP as a model of PNP. With analyzing the fluorescence intensities and TEM images of gFPNPs, we optimized the lyophilization process in terms of the process time, storage conditions, and compositions of stabilizers and finally evaluated the efficacy of lyophilization for the long-term storage of gFPNPs. We found an optimal condition that fluorescence and structure of gFPNPs stably maintained over 10 weeks, demonstrating that the key parameters for this stable and long-term maintenance of gFPNP can be applied in general to other PNPs. 2 Materials and methods 2.1 Gene expression and purification of gFPNPs The human ferritin heavy chain (hFTN-H) gene was cloned from a human liver cDNA library (Clontech, USA), and enhanced green fluorescent protein (eGFP) entire coding sequence was obtained by PCR using the plasmid pEGFP as a template. After PCR amplification using the appropriate primers and extention PCR, DNA fragments encoding each hFTN-H::Linker(G3SG3TG3SG3)::eGFP and eGFP were inserted into the Nde I -Hind III site of the pT7-7 plasmid by sequential ligation. The polyhistidine tag (His 6 ) was added to N-terminus of eGFP and C-terminus of FTN-H::Linker::eGFP (gFPNP) for metal (Ni 2+ ) affinity purification. The recombinant Escherichia coli strain BL21(DE3) [F − omp T hsd S B (rB − mB − )] was transformed with the plasmid expression vectors and cultivated at 37 °C with ampicillin resistant. When the optical density (OD 600 ) of the culture broth reached to 0.6–0.7, isopropyl- β - d -thiogalactopyranoside (IPTG) was added to broth with a final concentration of 1.0 mM. After inducing gene expression, the cells were further cultured at 20 °C for 16 h. The cells were then harvested by centrifugation (4500 rpm) for 10 min at 4 °C. The cell pellets were resuspended in 10 mL lysis buffer (50 mM sodium phosphate, 300 mM NaCl, 10 mM imidazole, pH 8.0) and then the solution was disrupted by sonification (Branson Ultrasonics Corp., CT, USA). The cell lysates was centrifuged at 13,000 rpm for 10 min and the soluble supernatant was obtained and subsequently loaded onto a Ni 2+ –NTA column (Qiagen, Germany). After sufficient washing with washing buffer (50 mM sodium phosphate, 300 mM NaCl, 50 mM imidazole, pH. 8.0), recombinant proteins were eluted using elution buffer (50 mM sodium phosphate, 300 mM NaCl, 250 mM imidazole, pH 8.0), and storage buffer was exchanged to PBS (137 mM NaCl, 2.7 mM KCl, 10 mM Na 2 HPO 4 , 2 mM KH 2 PO 4 , pH 7.4). 2.2 Lyophilization and rehydration of gFPNPs Three kinds of gFPNP samples (1.5 mg/mL) in PBS buffer were prepared without or with each stabilizer combination: 1 wt.% PEG and 5 wt.% sucrose; and 1 vol.% Tween 80 and 5 wt.% sucrose. Samples were slowly pre-freezed at −80 °C for 3 h and frozen samples were lyophilized at −80 °C in a freeze-dryer (FDU-2100, DRC-1000, EYELA, Japan). Primary drying was achieved at −10 °C for 1 h and secondary drying was achieved at 20 °C for 5 h in a vacuum. After that, Lyophilized samples were stored at different temperature conditions: −20 °C, 4 °C, 25 °C, and 37 °C. Each sample was completely sealed to inhibit the humidification. Lyophilized samples were rehydrated with deionized water. 2.3 Fluorescence intensity assay and transmission electron microscopy (TEM) for monitoring short- and long-term stability A fluorimetric microplate reader (GENios, Tecan, Austria) was used to measure the fluorescence intensity of eGFP and gFPNPs for short- and long-term stability. The concentration of the eGFP monomer and gFPNP samples was determined by Protein Assay Kit (Bio-Rad), and each sample was set to a final concentration of 10 pmol L −1 . About 50 μL of sample was added to the each well of 96-well plate (Cat. No. 137101, Nunc, Denmark). Filters for an excitation at 485 nm and emission at 535 nm were used. Fresh and rehydrated gFPNPs were imaged using a Philips Technai 120 kV electron microscope. Purified proteins were negatively stained with a 2 wt.% aqueous uranyl acetate solution. More detailed procedures for TEM image anlaysis of the purified protein nanoparticles were well described in previous report [3] . 3 Results and discussion 3.1 Stability of gFPNP in aqueous solution To evaluate the time-course variations in function and stability of gFPNP, we measured fluorescence intensities of gFPNPs stored at 4 °C every 2 day for 2 weeks and compared them with the fluorescence intensity of wild-type eGFP. As shown in Fig. 1 A, fluorescence intensity of gFPNP was maintained at more than 80% level of initial fluorescence, but that of eGFP decreased gradually to 20% of initial fluorescence. We also carried out TEM analysis to examine the structural stability of gFPNP and found that gFPNP retained a spherical nano-sized structure (10–15 nm in diameter) for 2 weeks ( Fig. 1 B and C). The results of Fig. 1 indicate that gFPNP can be actively maintained without denaturation of structure in aqueous solution (4 °C) even for 2 weeks. Reportedly, self-assembled particle structure of hFTN-H is remarkably stable and can be preserved even in high temperature and extreme pH, indicating that hFTN-H may be used as a template for stability enhancement against protein inactivation and/or denaturation [16,18] . Hence, the high structural stability of gFPNP results presumably from the outstanding stability of hFTN-H. 3.2 Lyophilization of gFPNP for long-term and stable maintenance Lyophilization was employed as a process to expand the maintenance of stable gFPNP up to 10 weeks, and diverse storage temperature conditions (−20 °C, 4 °C, 25 °C, and 37 °C) of lyophilized gFPNP were examined. Each gFPNP that was lyophilized without stabilizer was rehydrated in deionized water (DW), and the time-course fluorescence changes at each storage temperature were compared with the initial fluorescence of non-lyophilized gFPNP. For the first 4 weeks, fluorescence intensities of lyophilized gFPNPs stored at −20 °C and 4 °C were maintained up to more than 90% level of initial intensity ( Fig. 2 A and B), whereas those of lyophilized gFPNPs stored above room temperature (25 °C and 37 °C) decreased to about 60% level ( Fig. 2 C and D). To evaluate the correlation between the fluorescence and structural stability, we carried out the TEM analysis. After 4-week storage at −20 °C, lyophilized gFPNP still has almost the same spherical nanostructure as initial gFPNP ( Figs. 1B and 3A ). In the case of lyophilized gFPNP stored at 4 °C, the spherical nanoparticles of gFPNP were also observed although total number of nanoparticles significantly reduced ( Fig. 3 B). It seems that a simple dissociation of gFPNP particles occurred at 4 °C without aggregation, denaturation, and/or degradation of gFPNPs, so that the fluorescence emission was maintained without a significant decrease. On the other hand, almost all of lyophilized gFPNPs stored at 25 °C and 37 °C lost the unique nanostructure after 4 weeks due probably to significant denaturation of protein conformation, compared to initial gFPNPs ( Figs. 1B, 3C, and 3D ), i.e. lyophilized gFPNPs are severely denatured and inactivated as storage temperature increased. After 10-week storage at 25 °C and 37 °C, lyophilized gFPNPs without stabilizer lost their fluorescence to the 20% level of initial fluorescence ( Fig. 2 C and D). And also TEM images demonstrate that they completely lost the unique nanoparticle shape after 10 weeks ( Fig. 4 C and D), indicating that another approach to improve the lyophilization process (e.g. an addition of stabilizer) is required to enhance the long-term stability of gFPNPs. 3.3 Effect of Stabilizer on the long-term stability of lyophilized gFPNPs gFPNPs stored at −20 °C and 4 °C after lyophilized without stabilizer maintained their fluorescence and nanoparticle shape for 4 weeks, but after then they were gradually denatured and lost significantly the biological activity ( Figs. 2–4 ). To expand the period of maintaining the intrinsic functionality and structure, sucrose, Tween 80, and polyethylene glycol (PEG) were considered as stabilizer in the lyophilization of gFPNPs. These stabilizers are known to have different roles during lyophilization process: hydrogen bonding between sucrose and protein tends to protect the degradation of target proteins [19–22] ; PEG prevents the degradation and non-specific interactions between proteins during freezing process [20] ; and Tween 80 reduces the surface-induced unfolding of proteins during freezing-thawing process [20,22] . According to the report of Pikal-Cleland et al. [20] , however, neither PEG nor Tween 80 is effective as a stabilizer when each of them are used alone. And also it has been reported that combination of PEG-sucrose or Tween 80-sucrose is more effective than single use of sucrose [22] . Based on these backgrounds, we evaluated the efficacy of each stabilizer combination of PEG-sucrose and Tween 80-sucrose, to enhance the long-term stability of lyophilized gFPNPs at the same storage temperatures. As demonstrated in Fig. 2 , in the lyophilization processes using PEG-sucrose or Tween 80-sucrose, initial fluorescence intensities of lyophilized gFPNPs were stably maintained even for 10 weeks, except for the storage at 37 °C. We also investigated the changes in the structure/shape of gFPNPs through TEM image analysis and observed that unlike the stabilizer-free cases, the gFPNPs that were lyophilized with stabilizers retained their own spherical structure for 10 weeks ( Figs. 3 and 4 ), but the gFPNPs that were lyophilized in the presence of PEG-sucrose significantly lost its nanoparticle structure when stored at 25 °C or 37 °C ( Figs. 3L, 4K, and 4L ). PEG is a thermal sensitive polymer that has a low melting point about 50 °C [23] and seems to be not suitable as a stabilizer when the long-term storage following lyophilization undergoes at higher temperature above room temperature. Due to the small nanoscale size, gFPNPs have a high surface-to-volume ratio, implying that they have more chances to non-specifically interact with other gFPNPs. From Figs. 2–4 , it looks likely that Tween 80 that has a role to effectively prevent surface-induced unfolding of proteins reduces the interaction between gFPNPs during lyophilization, thereby resulting in enhanced maintenance of their own function and structure. Reportedly, several proteins including TNFβ, IL-1, bFGF, MDH, LDH, aldorase, and PFK were successfully lyophilized with Tween 80 and were protected from freezing-induced denaturation [15,22,24] . It is notable that the fluorescence of gFPNPs that were lyophilized with Tween 80-sucrose was retained at more than 80% level of initial fluorescence at every storage temperature for 10 weeks. This is due presumably to the effect of Tween 80 and/or synergy effect between Tween 80 and sucrose as well as the high stability of hFTN-H. In summary, protein nanoparticles (PNPs) are nanostructured biomaterials with intrinsic biological function and have been successfully employed in a wide range of applications such as sensitive bioassays, MRI contrast, semiconductor devices, template for hybrid materials, etc. To find the optimal conditions for enhancing and maintaining stability of PNPs, we examined the functional and structural stability of gFPNPs during a period of long-term maintenance. Prior to assessing the long-term stability, we analyzed the stability of gFPNPs in aqueous solution during a relatively short period and found that unlike fluorescent protein monomer (eGFP), they well retained fluorescence activity with maintaining their structural stability for 2 weeks when stored at 4 °C. To ensure stable and long-term maintenance of gFPNPs, we formulated gFPNPs into dried solid forms through lyophilization. The lyophilized gFPNPs were stably maintained for initial 4 weeks, but after then they significantly lost functional and structural stability without stabilizers. By adding stabilizers, especially both Tween 80 and sucrose, fluorescence and structure of lyophilized gFPNPs were successfully maintained up to 10 weeks without deformation/denaturation even at the storage temperature of 37 °C. Consequently, the lyophilization process employing Tween 80 and sucrose as stabilizers is an efficient and optimal method for the stable and long-term maintenance of gFPNPs with avoiding any structural and functional changes. Although this study was focused on gFPNPs, the developed lyophilization process/conditions can be applied generally to stable and long-term maintenance of many other PNP-derived nanoparticles. Acknowledgments This study was supported by the National Research Laboratory Project (Grant No. 2010-0018908 , the main project that supported this work), the Microbial Genomics and Applications Center at KRIBB (Grant No. 2010-K000599 ), the Public welfare & Safety Research Program (Grant No. 2010-0020778 ), and the Basic Science Research Program (ERC program, Grant No. 2010-0029409 ) of the National Research Foundation of Korea (NRF) grant funded by the Korea government. This work was also supported by the Second Brain Korea 21 Project, the Seoul R&BD Program (Grant No. PA100026M0211612 ), and an additional support from the National Research Foundation of Korea (NRF) (a Grant No. 2010-0027771 ). References [1] J.S. Park M.K. Cho E.J. Lee K.Y. Ahn K.E. Lee J.H. Jung Y. Cho S.S. Han Y.K. Kim J. Lee A highly sensitive and selective diagnostic assay based on virus nanoparticles Nat. Nanotechnol. 4 2009 259 264 [2] J.H. Lee J.S. Kim J.S. Park W. Lee K.E. Lee S.S. Han K.B. Lee J. Lee A three-dimensional and sensitive bioassay based on nanostructured quartz combined with viral nanoparticles Adv. Funct. Mater. 20 2010 2004 2009 [3] S.H. Lee H. Lee J.S. Park H. Choi K.Y. Han H.S. Seo K.Y. Ahn S.S. Han Y. Cho K.H. Lee J. Lee A novel approach to ultrasensitive diagnosis using supramolecular protein nanoparticles FASEB J. 21 2007 1324 1334 [4] J.M. Hooker A. Datta M. Botta K.N. Raymond M.B. Francis Magnetic resonance contrast agents from viral capsid shells: A comparison of exterior and interior cargo strategies Nano Lett. 7 2007 2207 2210 [5] L.O. Liepold M.J. Abedin E.D. Buckhouse J.A. Frank M.J. Young T. Douglas Supramolecular protein cage composite MR contrast agents with extremely efficient relaxivity properties Nano Lett. 9 2009 4520 4526 [6] A. Miura T. Hikono T. Matsumura H. Yano T. Hatayama Y. Uraoka T. Fuyuki S. Yoshii I. Yamashita Floating nanodot gate memory devices based on biomineralized inorganic nanodot array as a storage node Jpn. J. Appl. Phys. 45 2006 L1 L3 [7] A. Miura Y. Uraoka T. Fuyuki T. Yoshii I. Yamashita Floating nanodot gate memory fabrication with biomineralized nanodot as charge storage node J. Appl. Phys. 103 2008 074503 [8] T. Hikono T. Matsumura A. Miura Y. Uraoka T. Fuyuki M. Takeguchi S. Yoshii I. Yamashita Electron confinement in a metal nanodot monolayer embedded in silicon dioxide produced using ferritin protein Appl. Phys. Lett. 88 2006 023108 [9] C.E. Flynn S.W. Lee B.R. Peelle A.M. Belcher Viruses as vehicles for growth organization and assembly of materials Acta Mater. 51 2003 5867 5880 [10] P.G. Holder D.T. Finley N. Stephanopoulost R. Walton D.S. Clark M.B. Francis Dramatic thermal stability of virus-polymer conjugates in hydrophobic solvents Langmuir 26 2010 17383 17388 [11] M. Okuda K. Iwahori I. Yamashita H. Yoshimura Fabrication of nickel and chromium nanoparticles using the protein cage of apoferritin Biotechnol. Bioeng. 84 2003 187 194 [12] T. Douglas V.T. Stark Nanophase cobalt oxyhydroxide mineral synthesized within the protein cage of ferritin Inorg. Chem. 39 2000 1828 1830 [13] T. Douglas D.P.E. Dickson S. Betteridge J. Charnock C.D. Garner S. Mann Synthesis and structure of an iron(III) sulfide-ferritin bioinorganic nanocomposite Science 269 1995 54 57 [14] H.S. Seo S.E. Kim J.S. Park J.H. Lee K.Y. Yang H. Lee K.Y. Lee S.S. Han J. Lee A three-dimensional nanostructured array of protein nanoparticles Adv. Funct. Mater. 20 2010 4055 4061 [15] W. Wang Lyophilization and development of solid protein pharmaceuticals Int. J. Pharm. 203 2000 1 60 [16] J.S. Park J.Y. Ahn S.H. Lee H. Lee K.Y. Han H.S. Seo K.Y. Ahn B.H. Min S.J. Sim I.S. Choi Y.H. Kim J. Lee Enhanced stability of heterologous proteins by supramolecular self-assembly Appl. Microbiol. Biotechnol. 75 2007 347 355 [17] S. E. Kim, K. Y. Ahn, J. S. Park, K. R. Kim, K. E. Lee, S. S. Han, J. Lee, Anal. Chem. manuscript in preparation. [18] X. Yang P. Arosio D. Chasteen Molecular diffusion into ferrit pathways, temperature dependence, incubation time, and concentration effects Biophys J. 78 2000 2049 2059 [19] M.G. Anhorn H. Mahler K. Langer Freeze drying of human serum albumin (HAS) nanoparticles with different excipients Int. J. Pharm. 363 2008 162 169 [20] K.A. Pikal-Cleland J.F. Carpenter Lyophilization-induced protein denaturation in phosphate buffer systems: Monomeric and tetrameric β-Galactosidase J. Pharm. Sci. 90 2001 1255 1268 [21] H. Ohshima A. Miyagishima T. Kurita Y. Makino Y.Iwao.T. Sonobe S. Itai Freeze-dried nifedipine-lipid nanoparticles with long-term nano-dispersion stability after reconstitution Int. J. Pharm. 377 2009 180 184 [22] B.S. Chang B.S. Kendrick J.F. Carpenter Surface-induced denaturation of proteins during freezing and its inhibition by surfactants J. Pharm. Sci. 85 1996 1325 1330 [23] B. Perissutti, J. M. Newton, F. Podczeck, F. Rubessa, Preparation of extruded carbamazepine and PEG 4000 as a potential rapid release dosage form 2001, Eur. J. Pharm. Biopharm. 53 (2002) 125-132 [24] B. Kendrick B.Y. Chang J.F. Carpenter Detergent stabilization of proteins against surface and freezing denaturation Pharm. Res. 12 1995 S85
更多查看译文
关键词
Fluorescent protein nanoparticles,Lyophilization,Stability,Protein nanoparticle structure
AI 理解论文
溯源树
样例
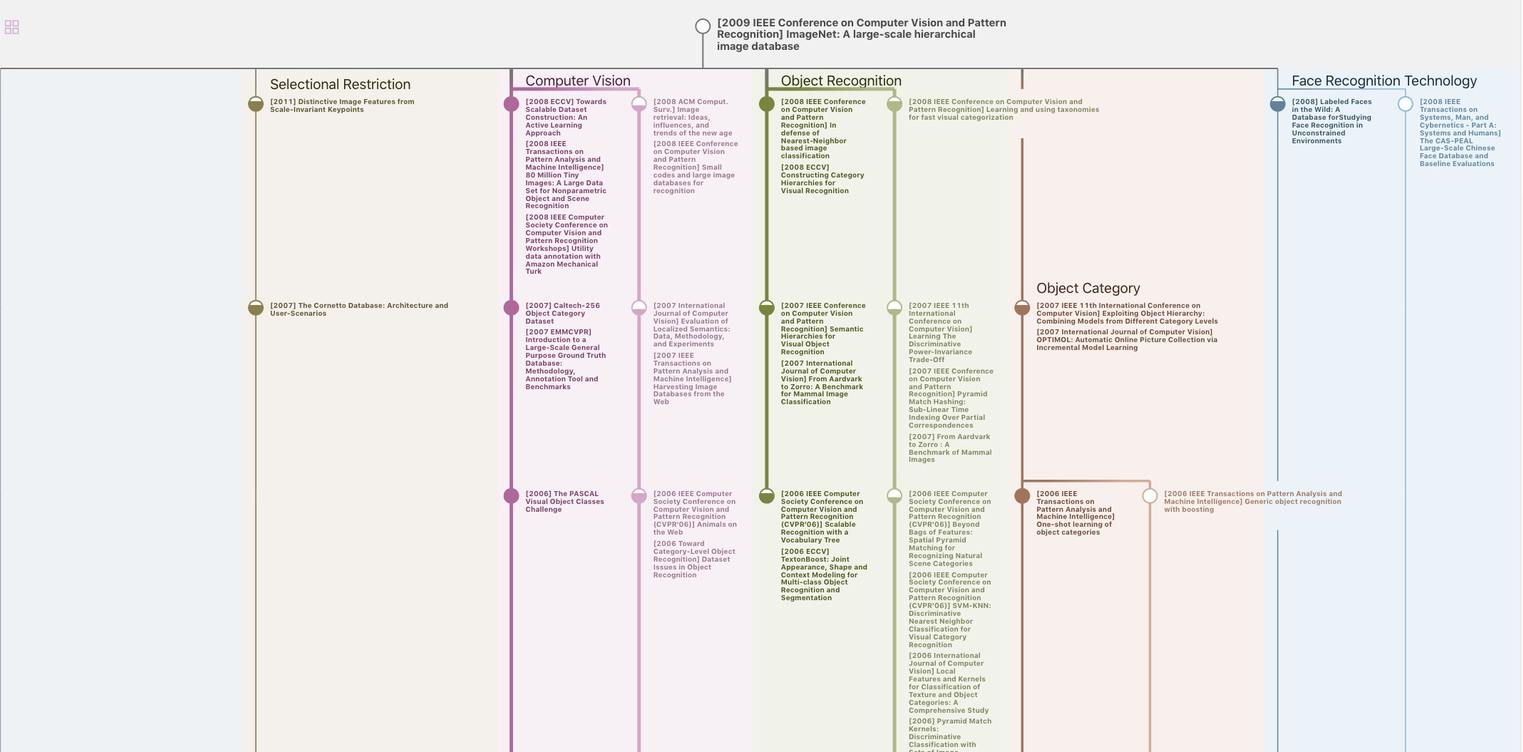
生成溯源树,研究论文发展脉络
Chat Paper
正在生成论文摘要