Comparison of IPSA with dose-point optimization and manual optimization for interstitial template brachytherapy for gynecologic cancers
Brachytherapy(2011)
摘要
Results Coverage of the clinical target volume (CTV) with GrO plan and IPSA algorithm was significantly better (mean V 100 of 88.8% and 89.1%; p = 0.006) as compared with DPO plan (83.7%; p = 0.62). Similarly, mean D 90 was same in both GrO plan and IPSA, 3.96 ± 0.23 and 3.96 ± 0.15 Gy, respectively. DPO plans were homogeneous with homogeneity index being 0.82 as compared with 0.68 ± 0.05 of GrO plan and 0.71 ± 0.04 of IPSA. However, IPSA resulted in high conformity with conformity index of 0.78 as compared with 0.72 ( p = 0.001) and 0.68 ( p ≤ 0.001) for GrO and DPO plans, respectively. The dose to rectum (3.3 ± 1.06 Gy) and bladder (3.17 ± 0.5 Gy) was generally high for DPO plan. GrO plan reduced the dose to the rectum (2.91 ± 0.63; p = 0.011) and bladder (2.89 ± 0.63 Gy; p = 0.003) significantly. IPSA resulted in a further reduction of the dose to rectum (2.79 ± 0.67 Gy; p = 0.046) and bladder (2.81 ± 0.67 Gy; p = 0.035), however with no statistical significance as compared with GrO plan. Conclusion IPSA resulted in significant sparing of normal tissues without compromising CTV coverage as compared with DPO plan. However, IPSA did not show any significant improvement either in CTV coverage or in normal tissue sparing as compared with GrO plan. IPSA was found to be superior in terms of homogeneity and conformity as compared with GrO plan. Keywords IPSA MUPIT Interstitial implant Gynecologic cancer Introduction Intracavitary brachytherapy plays a major role in the treatment of patients with cervical carcinoma. However, when pelvic anatomy is suboptimal because of narrow vagina or the absence of uterus postoperatively, interstitial brachytherapy using template plays an important role especially in advanced stage or recurrent diseases (1–6) . Various template systems have been developed for treatment of pelvic malignancies (7, 8) . Martinez universal perineal interstitial template (MUPIT) for brachytherapy was first devised by Martinez for prostate, cervix, vagina, female urethra, perineum, and anorectal region. The review of literature shows the evolution of this technique over the years, overcoming a number of limitations with advanced imaging modalities (9, 10) and dose optimization (11) . Three-dimensional treatment planning uses images of CT/MR to visualize target volumes and critical structures with respect to the implanted needles to produce customized dose distributions that may improve local control and reduce complications relative to conventional brachytherapy treatment planning (9, 10) . Furthermore, CT/MR imaging provides excellent soft tissue definition such that delineation of target and organs at risk (OARs) in relation to the applicator is possible. It enables to accurately assess the dose received by the target and the OARs. With the advances in imaging, the optimization methods also have improved. Graphical optimization, allows the user to change the dwell weights manually or drag the isodose lines using a mouse such that the target coverage is adequate with maximal sparing of OARs. It is a trial and error method where the planner has to keep changing the dwell weights until an optimal solution is met. It is quite time consuming and requires skill of an experienced planner. On the other hand, inverse optimization, (inverse planning simulated annealing [IPSA]), is based on a mathematical algorithm where clinical objectives are defined as mathematical equations. The optimal solution is obtained by minimizing the objective function through an iterative process. IPSA has been found to be superior with respect to target coverage and normal tissue sparing as compared with traditional optimization methods for prostate (12–17) , and gynecologic malignancies (11) . When IPSA was introduced in our clinic, a detailed study was carried out to commission the algorithm, which is the basis of this report. The purpose of this study is to objectively compare the dosimetric outcome of inverse planning algorithm as compared to dose-point optimized (DPO) plan and manual optimized plan/graphical optimization (GrO) plan. To our knowledge, ours is the first independent assessment of IPSA algorithm in a busy clinical practice for interstitial gynecologic malignancies using MUPIT. Methods and materials The data set of 10 consecutive patients of gynecologic cancers (post-op cervix: 3, vault recurrence: 4, and carcinoma vagina: 3) who underwent routine external radiotherapy and high–dose rate interstitial boost using MUPIT between September 2008 and October 2009 were selected for this dosimetric study. The external radiation dose was 50 Gy at the rate of 2.0 Gy/fraction followed by 20 Gy in 5 Gy/fraction of interstitial brachytherapy. The details of the brachytherapy procedure are described elsewhere (6) . To summarize, a pelvic examination under anesthesia was done followed by marking the boundaries of the residual disease using silver makers. The urinary bladder was catheterized with 7 mL of diluted urograffin pushed into its bulb, followed by the insertion of vaginal cylinder, the template then was placed and held to the perineum using 18 gauge needles with closed and trocar tips. Ultrasound imaging was used in a few patients as guidance for the placement of needles. Patients were then taken for imaging and planning. Dosimetry and optimization Image acquisition for treatment planning was done on the same day after the implant using axial CT scans of 3 mm slice thickness was taken on Somatom Emotion CT scanner (Siemens Medical Systems, Germany). The images were transferred to the Brachytherapy Planning System (PLATO-Sunrise v.14. 3, Nucletron B.V., Veenendaal, The Netherlands) via local area network. The dose computation algorithm in the treatment planning system is based on Task Group 43 as recommended by the American Association of Physicists in Medicine (18) . The radiation oncologist delineated the clinical target volume (CTV) using pretreatment clinical extent, imaging (pretreatment and post-EBRT), intraoperative findings, and radio-opaque silver markers placed during the procedure. The OAR’s, the rectum, bladder, and urethra were contoured. Reconstruction of the implant geometry was carried out using multiplanar reconstruction. This algorithm enables the planner to track the catheters in axial, coronal, and sagittal planes. A negative offset of 9 mm was given for each needle to compensate for the dead space (5.5 mm) and the source clearance (3.5 mm). The dwell positions were loaded based on the CTV, with an extra margin of 5 mm across the CTV. For each patient three plans were generated. DPO plan The dose points were placed between the catheters in accordance with the stepping source dosimetry system throughout the CTV. The dose points were created using the option of creating basal points in the treatment planning system. In our case, generally square geometry was used. The plans were dose-point optimized on volume. The dose was prescribed on the dose points. The prescription isodose line was 85–100% line, variable from patient to patient based on the coverage. GrO plan The DPO plans were manually optimized, wherein the active dwell weights were changed. This could be done by clicking the isodose line with the mouse dragging it to the desired location. Alternately, the dwell weights of those positions contributing to the dose to the OARs were reduced and the dwell weight of those positions contributing to the CTV coverage was increased. This was done repeatedly until a satisfactory plan was obtained with respect to CTV coverage, sparing of OARs, homogeneity, and conformity in that order. The plan objective for optimization was to maximize the CTV coverage, whereas minimizing the dose to the OARs. Because GrO/manual forward planning is operator dependent, to maintain uniformity among the manually optimized plans, emphasis was given to adequately cover the CTV, while trying to reduce the dose to the OARs to the predetermined dose objective parameters. The dose objective parameters for the OARs were as follows. Dose to the most exposed volume, D 2cc of bladder and rectum was 3.0 and 2.5 Gy, respectively whereas D 1cc of urethra was 3.0 Gy. For CTV, dose to 90%, D 90 of 4 Gy was considered. Inverse plan IPSA was used to generate an inverse plan, where the dwell positions were maintained similar to DPO plans. The plan objectives were similar to that of GrO plan. The relative importance factors and the dose constrains were changed until an optimal plan was obtained that meets the dose objective parameters of both CTV and the OARs. Table 1 provides a representative set of dose objective parameters used to obtain an IPSA plan. Plan evaluation Dosimetric outcome from these three different plans were compared. For quantitative analysis, cumulative dose–volume histogram (DVH) was calculated for every plan with 25 mm margins around the implanted volume in all directions with 100,000 calculation points randomly placed in the volume of interest. For the CTV, D 90 , the dose covering 90% of the CTV volume, and V 100 , the percentage volume covered by 100% of the CTV volume were evaluated. V 200 , the absolute volume covered by 200% of the prescription isodose, was evaluated. For OARs, D 2cc , minimal dose received by the most irradiated 2 cc volume of bladder and rectum were compiled (19) . For urethra, dose to 1 cc volume was considered. In addition, V 75 , the volume receiving 75% of the prescription dose was evaluated for rectum, bladder, and urethra. Dose–volume indices, including homogeneity index (HI) and conformity number were evaluated (20) . HI is the fraction of CTV receiving a dose between 100% and 150% of the reference dose. To evaluate the statistical significance, we made an analysis of the descriptive statistics (mean, standard deviation, and 95% confidence interval) of dosimetric parameters for the three optimization techniques. The parameters were compared for the three groups using paired t test. The level of significance was set at 0.05. Results All 10 applications were evaluable for this dosimetric outcome. The average number of implanted needles was 23 ± 6 (range, 18–25). The dose distribution of DPO, GrO, and IPSA plans of a representative patient is shown in Fig. 1 . Table 2 illustrates the dose–volume parameters of all the plans. The mean (standard deviation) volume of CTV was 123.97 ± 40.57 cc (range, 68.04–190.48 cc). The mean volume of rectum and bladder was 43.76 ± 18 and 121.05 ± 59.18 cc, respectively. Coverage of the CTV with GrO and IPSA was significantly better (mean V 100 of 88.8% and 89.1%; p = 0.006) as compared with DPO plan (83.7%). Similarly, mean D 90 was same in both GrO and IPSA plan, namely, 3.96 ± 0.23 and 3.96 ± 0.15 Gy, respectively. Although both plans have resulted in similar D 90 , it was observed that IPSA plans were more reproducible as compared with GrO plan. This could be observed from the standard deviation of the IPSA plan, 0.15 Gy as compared with GrO plan 0.23 Gy ( p = 0.003). Mean volume receiving 150% of dose was 25.6% and 26.9% for GrO and IPSA as compared with 15.3% of DPO plan, which is reflected in HI. DPO plans were more homogeneous with HI being 0.82 as compared with 0.68 ± 0.05 of GrO and 0.71 ± 0.04 of IPSA. However, IPSA plans resulted in high conformality with conformity index of 0.78 as compared with 0.72 ( p = 0.001) and 0.68 ( p ≤ 0.001) for GrO and DPO, respectively. Total reference air kerma of DPO and IPSA plans were 0.27 ± 0.06 cGy, whereas for GrO it was 0.29 ± 0.06 cGy. Figure 2 a–c illustrates the relation between the dose to the OAR ( D 2cc ) with respect to CTV coverage ( D 90 ), for DPO, GrO, and IPSA plan. The lower right quadrant represents the dose limits of the OAR without compromising the CTV coverage. It could be seen that rectum is the dose-limiting factor ( Fig. 2 a), and none of the DPO plans could satisfy the dose-limiting criteria. For IPSA and manual optimization, it was observed that most of the patients were approaching toward the lower right quadrant, which confirms that optimization plays a major role in reducing the dose to the OARs, while maintaining CTV coverage. The dose to rectum ( D 2cc : 3.3 ± 1.06 Gy) and bladder (D 2cc : 3.17 ± 0.5 Gy) was generally high for DPO plans. However, dose to urethra (D 1cc : 2.53 ± 0.88) was well within the tolerance limits. GrO reduced the dose to the rectum (2.91 ± 0.63 Gy; p = 0.011) and bladder (2.89 ± 0.63 Gy; p = 0.003) significantly. IPSA resulted in a further reduction of the dose to the rectum (2.79 ± 0.67 Gy; p = 0.046) and bladder (2.81 ± 0.67 Gy; p = 0.035), however with no statistical significance as compared with GrO. Figure 3 shows the individual dwell time of catheters for DPO, GrO, and IPSA plans of a representative patient. DPO plans showed a smooth variation between the dwell time in the catheters, which resulted in highly homogeneous plans. On the other hand, both IPSA and GrO plans resulted in steep variation in dwell time, which could be seen from the DVH. The long tail in the DVH of CTV represents the low volumes of high dose regions ( Fig. 4 ). It was observed that in DPO, none of the dwell times were completely turned off even if the dwell position is next to a critical structure; however, in IPSA and in GrO the dwell time next to a critical structure were turned off, whereas long dwell times were noticed where no critical structures were present. The optimization time for DPO plan was 1–2 min, whereas planning time for IPSA was about 10 min. Changing the constraints and reevaluating the dose–volume parameters to arrive at an optimal plan was indeed a time consuming factor for IPSA plans too. Each GrO plan had taken about 15–20 min to arrive at an optimal plan by an experienced physicist. Changing the individual dwell weights or manually dragging the isodose lines using a mouse and evaluation of dose–volume parameters was a time consuming process during GrO plans. Discussion The results of the present study have shown that IPSA has resulted in superior plans in terms of CTV coverage, sparing normal tissues and conformality as compared with DPO algorithm. However, this benefit came with a decrease in homogeneity. In our study, DPO plans were highly homogeneous; however, IPSA plans were associated with reduced HI. This is the only difference observed between the present study and the earlier investigations comparing IPSA with other algorithms, such as geometric optimization, DPO, and GRO (13, 17, 21, 22) . Most of the earlier investigations were carried out in prostate, using template technique (13, 17, 21, 22) . Although the site is different, we expected the results to be similar because of the similar implantation technique and dose calculation algorithm. The reduction of homogeneity in IPSA plans may be attributed to the geometry of the implant, where the needles are placed laterally to cover the parametrial region, where as in prostate implants, the needles are positioned in a circular fashion. Moreover, in gynecologic implants, achieving a good geometry was limited with the usage of diverging needles to avoid the converging of needles in the parametrium laterally, which may distort the geometry of the implant and in turn homogeneity. Diverging needles are also used in a situation where the implant pathways are obstructed because of pubic bones ( Fig. 5 ). Another reason could be large CTV volumes associated with the gynecologic implants as compared with prostate implants. The mean CTV volume in the present study was 124 cc, whereas the prostate volumes reported in the literature has a mean CTV volume of the order of 30–50 cc (13, 21) , except Lachance et al. (14) who reported large prostate volume of 102.84 cc. Hence, our results did not compare well with other studies of IPSA with regard to homogeneity; however, with regard to CTV coverage and OAR sparing, it agrees well with the others (13, 17, 21, 22) . It is unclear whether dose homogeneity is a necessity in gynecologic implants, as long as the CTV is covered adequately with optimal sparing of OARs. However, it may be a cause of concern especially during dose escalation and simultaneous boost. Vikram et al. (23) reported that the relationship between the hot spot and the complication rate is insignificant for high–dose rate interstitial brachytherapy implants, so the hotspots need not preclude optimization to ensure adequate coverage of the CTV. Hence, reduction of HI is acceptable during inverse and graphical optimization for adequate CTV coverage. In some implants, it was observed that because of large geometrical irregularities, the HI becomes reduced (<0.65). In another publication, Wazer et al. (24) reported that the size of high-dose regions correlates to necrosis for breast implants, and the reduction of high-dose volumes may reduce the normal tissue complication. Although there is limited data available correlating the hot spots and its consequences for gynecologic implants, the use of inverse and graphical optimization to obtain good CTV coverage in implants with geometrical irregularities has to be done with caution (25) . It was also observed that the coverage was significantly low in DPO as compared with GrO and IPSA plan, which could be attributed to the location of the dose points. In our case, we have positioned the dose points inside the CTV and used a prescription isodose of 85–100%, which sufficiently covers the CTV. Alternatively, the dose points could be positioned around the CTV about 3–7 mm, in such a case the coverage may be superior, however, with an increased dose to the OARs, which may be partially rectified by positioning the dose points only laterally and not anterioposteriorly or by differentially weighting or prescribing the dose points. GrO is a powerful tool as it provides an interface, which allows the planner to drag isodoses on the computer screen to the desired position. In the absence of inverse planning softwares, we had routinely used GrO for our implants and found these plans to be clinically acceptable, providing optimal sparing of OARs, while maintaining adequate CTV coverage. It is worth mentioning here that the planning process of GrO requires skill and experience from the planner point of view. It is laborious and time consuming as compared with other optimization algorithms. At sometimes, unacceptably large amount of hot spots may occur at some cross section, which can go unnoticed even by DVH analysis. Evaluation of isodose distribution in each cross section, DVH analysis, and evaluation of quality indices may be warranted in arriving at an optimal plan when using GrO. Even an experienced planner can take about 20–30 min to arrive at an optimal plan, whereas IPSA can be generated in 5–10 min. Another advantage of IPSA is that once a class solution is made, it can be recalled for every patient that reduces the planning time; on the other hand, GrO plan is patient-specific, which has to be obtained by individually looking at CTV coverage and organ sparing at the same time. Although GrO produces clinically acceptable plans that are comparable with IPSA, attempts at graphical optimization should be undertaken with a little caution keeping in mind all the possible variations of isodose distributions resulting from the GrO. Conclusion IPSA resulted in significant sparing of normal tissues without compromising CTV coverage as compared with DPO. However, IPSA did not show any significant improvement either in CTV coverage or in normal tissue sparing as compared with GrO. IPSA plans were found to be superior in terms of homogeneity and conformity as compared with GrO. References [1] A. Eisbruch C.M. Johnston M.K. Martel Customized gynecologic interstitial implants: CT-based planning, dose evaluation, and optimization aided by laparotomy Int J Radiat Oncol Biol Phys 40 1998 1087 1093 [2] B. Erickson K. Albano M. Gillin CT-guided interstitial implantation of gynecologic malignancies Int J Radiat Oncol Biol Phys 36 1996 699 709 [3] S. Beriwal A. Bhatnagar D.E. Heron High-dose-rate interstitial brachytherapy for gynecologic malignancies Brachytherapy 5 2006 218 222 [4] K.G. Anjali A.V. Frank J.F. Arthur Iridium-192 transperineal interstitial brachytherapy for locally advanced or recurrent gynecological malignancies Int J Radiat Oncol Biol Phys 43 1999 1055 1060 [5] S. Nag M.R. Martinez R. Ellis The use of fluoroscopy to guide needle placement in interstitial gynecological brachytherapy Int J Radiat Oncol Biol Phys 40 1998 415 420 [6] R. Susworo N. Supriana I. Ramli HDR interstitial perineal implant for locally advanced or recurrent uterine cervix cancer Radiat Med 22 2004 2 5 [7] A.M.N. Syed D. Neblett B.H. Feder Treatment of extensive carcinoma of cervix with the “transperineal parametrial butterfly” Int J Radiat Oncol Biol Phys 4 1978 735 742 [8] A. Martinez R.S. Cox G.K. Edmundson A multiple site perineal applicator (MUPIT) for treatment of prostatic, anorectal, and gynecologic malignancies Int J Radiat Oncol Biol Phys 10 1984 297 305 [9] A.N. Vishwanathan R. Cormack C.L. Holloway Magnetic resonance guided interstitial therapy for vaginal recurrence of endometrial cancer Int J Radiat Oncol Biol Phys 66 2006 91 99 [10] K. Yoshida H. Yamazaki T. Takenaka A dose volume analysis of magnetic resonance imaging-aided high-dose-rate image-based interstitial brachytherapy for uterine cervical cancer Int J Radiat Oncol Biol Phys 77 2009 765 772 [11] E. Lessard I.C. Hsu J. Pauliot Inverse planning for interstitial gynecologic template brachytherapy: Truly anatomy-based planning Int J Radiat Oncol Biol Phys 54 2002 1243 1251 [12] J. Pouliot E. Lessard I.C. Hsu Advanced 3D planning B.R. Thomadsen M.J. Rivard W.M. Butler Brachytherapy physics. AAPM Medical Physics Monograph No.31 2005 Medical Physics Publishing Madison, WI 393 414 [13] I.C. Hsu E. Lessard V. Weinberg Comparison of inverse planning simulated annealing and geometrical optimization for prostate high dose rate brachytherapy Brachytherapy 3 2004 147 152 [14] B. Lachance D. Beliveau-Nadeau E. Lessard Early clinical experience with anatomy based inverse planning dose optimization for high dose rate boost of the prostate Int J Radiat Oncol Biol Phys 54 2002 86 100 [15] Y. Yoshioka T. Nishimura M. Kamata Evaluation of anatomy based dwell position and inverse optimization in high dose rate brachytherapy of prostate cancer: A dosimetric comparison to a conventional cylindrical dwell position, geometric optimization and dose point optimization Radiother Oncol 75 2005 311 317 [16] I.K. Kolkman-Deurloo X.G. Deleye P.P. Jansen Anatomy based inverse planning in HDR prostate brachytherapy Radiother Oncol 73 2004 73 77 [17] D. Jacob A. Raben A. Sarkar Anatomy-based inverse planning simulated annealing optimization in high-dose-rate prostate brachytherapy: Significant dosimetric advantage over other optimization techniques Int J Radiat Oncol Biol Phys 72 2008 820 827 [18] R. Nath L.L. Anderson G. Luxton Dosimetry of interstitial brachytherapy sources: Recommendations of the AAPM Radiation Therapy Committee Task Group no 43 Med Phys 22 1995 209 234 [19] C. Kirisits R. Potter S. Lang Dose and volume parameters for MRI based treatment planning in intracavitary brachytherapy for cervical cancer Int J Radiat Oncol Biol Phys 62 2005 901 911 [20] A. Van’t Riet A.C. Mak M.A. Moerland A conformation number to quantify the degree of conformality in brachytherapy and external beam irradiation: Application to the prostate Int J Radiat Oncol Biol Phys 37 1997 731 736 [21] G.C. Morton R. Sankreacha P. Halina A comparison of anatomy-based inverse planning with simulated annealing and graphical optimization for high-dose-rate prostate brachytherapy Brachytherapy 7 2008 12 16 [22] A. Mahmoudieh C. Tremblay L. Beaulieu Anatomy-based inverse planning dose optimization in HDR prostate implant: A toxicity study Radiother Oncol 75 2005 318 324 [23] B. Vikram S. Deore J.J. Beitler The relationship between dose heterogeneity (hot spots) and complications following high dose rate brachytherapy Int J Radiat Oncol Biol Phys 43 1999 983 987 [24] D.E. Wazer D. Lowther T. Boyle Clinically evident fat necrosis in women treated with high-dose-rate brachytherapy alone for early-stage breast cancer Int J Radiat Oncol Biol Phys 50 2001 107 111 [25] B.R. Thomadsen S. Shahabi D. Buchler Differential loadings of brachytherapy templates Endocurietherapy/Hyperthermia Oncology 6 1990 197 202
更多查看译文
关键词
IPSA,MUPIT,Interstitial implant,Gynecologic cancer
AI 理解论文
溯源树
样例
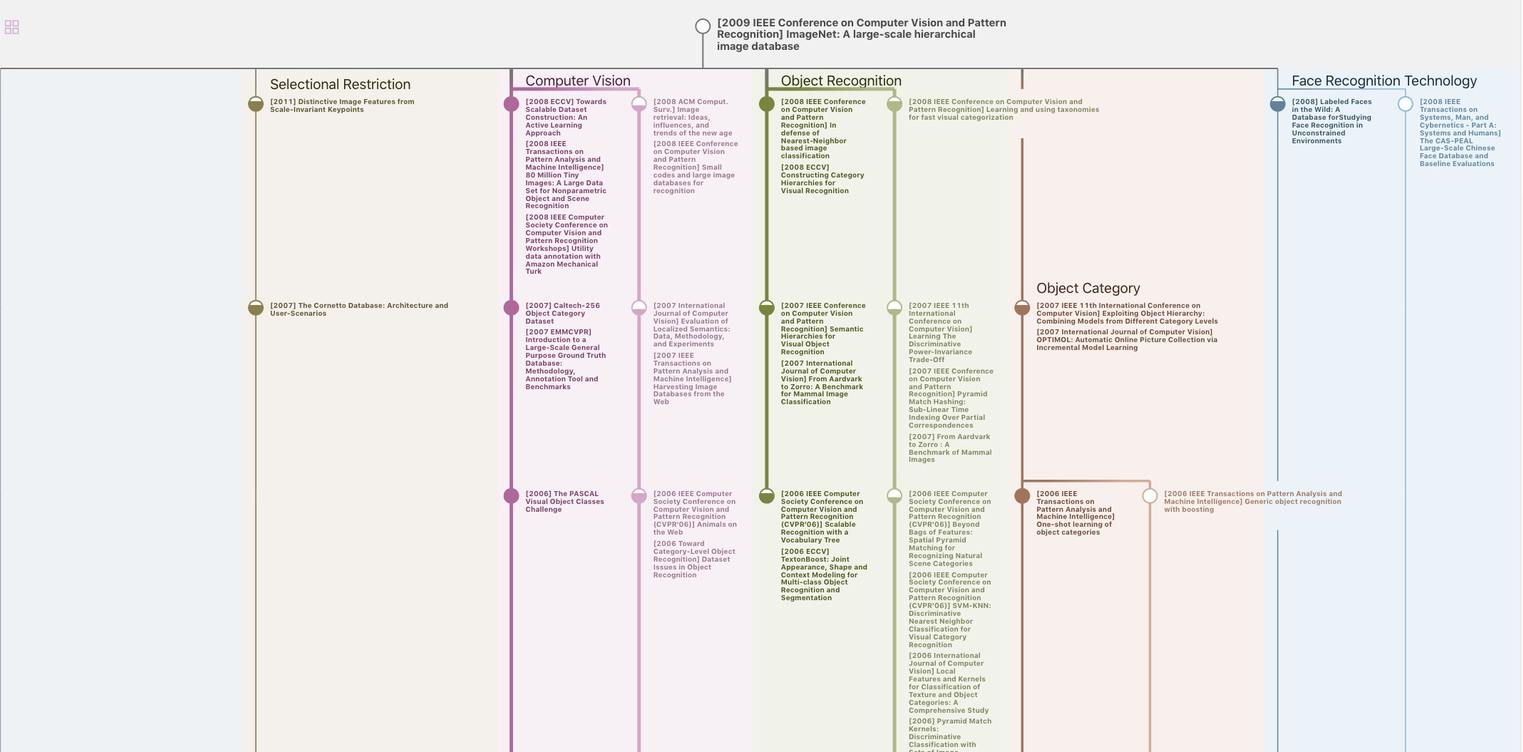
生成溯源树,研究论文发展脉络
Chat Paper
正在生成论文摘要