Automated FTIR Analysis of Free Fatty Acids or Moisture in Edible Oils
Journal of the Association for Laboratory Automation(2006)
摘要
An FTIR spectrometer coupled to an autosampler and attendant methodologies for high-volume automated quantitative analysis of free fatty acids (FFA) or moisture in edible oils are described. Samples are prepared by adding 20 g of oil to a 50 ml screw-capped vial, to which is added either a methanol/NaHNCN solution or dry acetonitrile in a 1:1 (w/v) ratio for FFA or H 2 O analysis, respectively. After capping with Mylar-lined septum caps, the vials are loaded into an autosampler tray, which is then agitated vigorously to extract the constituent of interest from the oil into the oil-immiscible solvent, and are then left to stand for ∼10 min to allow for phase separation. The upper solvent layer in each vial is aspirated successively into the IR cell, with the Mylar seal allowing facile autosampler needle penetration into the vials. The spectra of the sample extraction solvents serve as spectral backgrounds in addition to being used in monitoring cell path length and verifying cell loading. FFA and H 2 O analyses are carried out using 100 and 500 μm CaF 2 cells, respectively. For FFA analysis, quantification is achieved using the ν (COO − ) band at 1573 cm −1 , while moisture is determined using water absorption bands at 3629 or 1631 cm −1 , depending on the moisture range of the samples. Calibration procedures and data are presented. The spectrometer and autosampler are controlled using proprietary Universal Method Platform for InfraRed Evaluation software, which provides a simple user interface and automates the spectral analysis; the output data can also be sent to a Laboratory Information Management System. Validation and performance data obtained with this automated system demonstrate that it is capable of analyzing >60 samples/h, a rate commensurate with the throughput required by commercial contract or high-volume process control laboratories. Keywords free fatty acids water edible oils FTIR automation Introduction Edible oils represent one of the primary constituents in the formulation and manufacture of products by the food industry. Oils are extracted from a wide range of raw materials and generally undergo relatively standardized extraction and refining processes and supplemental transformations such as fractionation, hydrogenation, and/or interesterification prior to use. During processing, a variety of physicochemical oil quality parameters are monitored, including the determination of free fatty acids (FFA), moisture (H 2 O), peroxide value, iodine value, and saponification number to name just a few. 1 Typically, QC laboratories have been limited to using standardized American Oil Chemists' Society (AOCS 2 ) or International Union of Pure and Applied Chemistry (IUPAC 3 ) wet chemical methods to monitor oils in process, requiring a variety of analytical setups and multiple reagents as well as skilled personnel to carry them out, although these methods have been automated to some extent through the use of automatic titrators and flow injection systems. The McGill IR Group has worked toward developing FTIR spectroscopy as a rapid instrumental technique for the routine analysis of edible oils. Methodologies have been developed for the determination of FFA, 4,5 saponification number, 6 iodine value, 6,7 trans and cis content, 7–9 ansidine value, 10 solid fat index, 11 and peroxide value, 12–14 amongst others. From the standpoint of implementation, most of these methods are semiautomated, with the software providing a simple user interface in addition to controlling the instrument, performing all calculations, and storing the results automatically. These FTIR methods provide improved sample turnaround and a reduction in reagent use relative to wet chemical methods. However, they do not provide the level of speed required by contract or centralized QC laboratories handling high-sample volumes; the key factor limiting sample throughput being the high viscosity of edible oils. On the other hand, in the case of new FTIR methods that have recently been developed in our laboratory for the determination of FFA 15 and H 2 O 16 in edible oils, this limitation is obviated because the constituent of interest is extracted into a low-viscosity solvent prior to FTIR analysis. Indeed, these new FTIR methods have the potential for much higher sample throughputs than autotitration systems, which presently provide the most rapid means of obtaining reliable FFA or moisture data. In addition to reducing the sample-flow constraints associated with high-viscosity oils, these extraction-based methods largely eliminate problematic matrix effects and provide much greater sensitivity than can generally be achieved by direct FTIR analysis of oils. This paper describes the instrumentation, analytical protocols, and software associated with an automated FTIR/autosampler system designed to analyze of either FFA or H 2 O in edible oils at rates of up to 100 samples per hour. Methodology Instrumentation The analytical system used couples a Bomem WorkIR (ABB Bomem, Quebec City, PQ, Canada) FTIR spectrometer with a Model 223 Gilson autosampler (Gilson, Inc., Middleton, WI) and a positive displacement micropump (Vissers Sales Corp., Aurora, Ont., Canada). The spectrometer is equipped with a deuterated triglycine sulfate (DTGS) detector and uses 100 and 500 μm CaF 2 transmission flow cells (International Crystal Laboratories, Garfield, NJ) for FFA and H 2 O analyses, respectively, with Luer-Lock cell connectors used to facilitate cell changes. Software The spectrometer, micropump, and autosampler were all controlled by an IBM-compatible PC running proprietary Windows-based Universal Method Platform for InfraRed Evaluation (UMPIRE Pro) software, developed by Thermal-Lube Inc. (Pointe-Claire, PQ, Canada). Built on the Microsoft.NET Framework, UMPIRE Pro software is designed to work with Windows XP or Windows 2000 operating systems. UMPIRE Pro is a self-contained platform that allows for the acquisition of spectra, analysis of spectral data, and cataloguing of results without operator intervention. For systems equipped with an autosampler and pump (such as that used in the present work), the analysis of all the samples loaded into the autosampler tray is fully automated. All parameters associated with the three fundamental tasks controlled by the software (spectral acquisition, data processing, and cataloguing of results) are predefined for each type of analysis (e.g., FFA determination, H 2 O determination) in an “Analysis Method”; additional customized Analysis Methods can be created using the method development tool provided in the software. The Analysis Method specifies all relevant acquisition parameters relating to the spectrometer and the autosampler, the component(s) to be quantified, and the “Component Method” associated with each component, where the latter specifies the spectral features that will be used for its quantification and the calibration (linear or polynomial) by which the results will be computed. All results are archived within the software and can be retrieved by referencing the autosampler tray, analysis method, operator who acquired the samples, value of result, etc. These results can be printed, exported to external software for further analysis or reporting, or reprocessed by a different analysis method. Through the use of eXtensible Markup Language and the Microsoft.NET environment, UMPIRE Pro can relay information to a Laboratory Information Management System (LIMS). Reagents All solvents were of HPLC grade, obtained from Fisher Scientific (St. Louis, MO). Acetonitrile was dried over 4–8 mesh 4 Å molecular sieves for a minimum of 2 weeks and dispensed using a repipette (Hirschmann-Laborgerate, Germany) protected by Drierite to prevent moisture ingress. 16 The reagent solution used in the FFA analysis was prepared by dissolving NaHNCN in MeOH (2 g/L). Before use, this solution was allowed to stand for ∼4 days or until the ν (C N) band at 2100 cm −1 completely disappeared. 15 Both extraction solvents (acetonitrile and methanol/NaHNCN) were kept in sealed bottles and maintained over molecular sieves. Calibration Standards For the FFA calibration, standards were prepared by adding palmitic acid (0–1%) to canola oil, which had been passed through an activated silica gel column to remove any traces of FFA and other oxygenated compounds. Moisture calibration standards were prepared by gravimetric addition of distilled water (0–1000 ppm) to corn oil, which had previously been kept over 10–20 mesh Drierite (calcium sulfate) for at least 1 week. Analytical Protocol Both the FFA and moisture analyses are based on the extraction of the constituent of interest into an oil-immiscible solvent. For the analysis of FFA, the extraction uses methanol containing a weak base, sodium hydrogen cyanamide (NaHNCN), the latter producing immediate conversion of acids into methanol-soluble salts without causing saponification of the oils, whereas for moisture analysis the sample is extracted with dry acetonitrile. The details associated with the procedures for these analyses have been described elsewhere. 15,16 Figure 1 presents a generalized schematic diagram of the analytical protocol followed for both the calibration standards and the samples. For either FFA or moisture analysis, 20 g of oil was weighed into a tared 50-mL plastic vial to within ∼±0.10 g. To the vial containing oil, 20 ml of the appropriate solvent was added using a calibrated propipette, and the vial was capped. The vials were agitated on a vortex mixer for 30 s and then loaded onto the autosampler tray. The thoroughly mixed samples were then left to stand for 10–15 min to ensure separation of the oil and solvent layers. The automated FTIR spectral collection procedure was initiated with the collection of an open-beam spectrum followed by a single-beam solvent spectrum, the latter ratioed against the former to produce a reference solvent spectrum used to determine cell path length. The single-beam spectrum of the solvent layer in each sample vial was then recorded and ratioed against the single-beam solvent spectrum to obtain the spectrum of the constituent being analyzed for; the same single-beam solvent spectrum was used for a complete autosampler tray (56 samples). All spectra were collected by co-adding 32 scans at a resolution of 8 cm −1 and a gain of 1.0. For FFA analysis, the absorbance of the ν (COO − ) band at 1573 cm −1 relative to a baseline of 1820 cm −1 was measured and the FFA content predicted from a calibration equation obtained by regressing the corresponding absorbance values of the FFA calibration standards against their FFA content, expressed as % FFA (oleic acid). For moisture analysis, the calibration equations used were based on relating the amount of moisture added to the moisture standards to the absorbance of the strong ν (O–H) band at 3629 cm −1 and/or the weaker δ (H–O–H) band at 1631 cm −1 , both measured relative to a single-point baseline at 2500 cm −1 . We have established empirically that measurement of the more sensitive 3629 cm −1 band is optimal up to a moisture level of 2000 ppm for a 500 μm cell, beyond which the 1631 cm −1 band is preferred. The 2000 ppm limit corresponds to an absorbance at 3629 cm −1 of ∼0.7, and the switchover from the 3629 to the 1631 cm −1 calibration equation is automatically made by the software based on this absorbance criterion. System Evaluation and Validation Optimization of the analytical system in terms of minimizing sample carryover was initially evaluated by sequentially analyzing low ( L ) and high ( H ) FFA or moisture standards and calculating percent carryover as [( L 2 − L 1)/ H 1] × 100. Sample carryover was further assessed by analyzing three replicates of six different oils of unknown FFA or H 2 O content, these 18 samples being placed in the autosampler tray in a random order. Subsequently, reproducibility and accuracy of the optimized automated FTIR method were assessed by standard addition of palmitic acid or water to olive, sunflower, and peanut oils, each validation sample being analyzed in duplicate, on different days, by the appropriate FTIR method and the results compared to the gravimetrically added amounts of these constituents. Further validation of the FFA method was performed with 50 pre-analyzed production samples obtained from a major corn oil processor (Casco Inc.); similar pre-analyzed samples for moisture content were not available to us. Results and discussion System Overview and Software Figure 2 illustrates the overall system configuration. Because the FFA and moisture methods are designed to analyze low-viscosity solvent extracts rather than oils per se, a small micropump can be used to aspirate the sample into the IR cell. Low dead volume combined with 1/8 in. i.d. tubing minimizes sample volume (∼15 ml required) and allows the elimination of solvent rinses between samples. The sample vials have conventional plastic septum caps but the silicone septum is replaced by a 20 mil Mylar liner. This Mylar liner provides the hermetic seal required for moisture analysis while being readily punched through by the autosampler needle ( Fig. 3 ). Both the FTIR spectrometer and the autosampler are controlled by UMPIRE Pro, an upgrade of a proprietary software package designed as a general platform for developing and implementing FTIR methods. Details regarding the design of the software are presented in the Methodology section, and some features of the user interface are illustrated in the panels of Figure 4 . The user interface consists of a nested set of tab-based menus that allow the user to implement calibration and method development procedures. Figure 4 A presents the “General” descriptive tab associated with the FFA method, and Figure 4 B presents the Scanning/Sampling tab, from which the FTIR operating parameters and autosampler/pump parameters can be input. Figure 4 C illustrates the typical tabs included under the “Components” submenu, showing the tabs that can be accessed from this submenu to define the peak height or area measurements to be used for quantification and input calibration equations, warning messages for the operator, alternate calibration equations to be used under defined conditions, and baseline correction procedures. This tab-based user interface makes it simple and intuitive to develop, assess, and modify methods as well as calibration procedures. In addition to these development and setup functions, spectra can be viewed, manipulated, and exported, and the analytical result(s) can be displayed, printed, trended, and/or associated with a client, machine, or operation as well as output to an LIMS for further processing or reporting. For the present work, the autosampler was equipped with a 56-slot (7 × 8) tray. The first two slots of the autosampler tray were reserved for the solvent used for the analysis in question, the spectrum of which not only served as a background spectrum but was also used to determine the cell path length. For the latter purpose, a path length calibration equation was developed by building three cells of different path lengths and relating their path lengths, as determined from the fringe count in the spectrum of the empty cell, to the height of an on-scale solvent band. Typical linear regression equations obtained for acetonitrile and methanol, respectively, were (1) Pathlength ( μ m ) = 673.51 × A 2627 / 2580 cm − 1 − 18.488 R 2 = 0.999 SD = 1.75 (2) Pathlength ( μ m ) = 261.99 × A 2044 / 1952 cm − 1 − 0.251 R 2 = 0.999 SD = 0.384 Using these equations, a cell path length is determined at the start of each run, of which an ongoing record is maintained to monitor the condition of the cell over time. This path length value is used to normalize the spectral data subsequently collected for the samples in the tray to a constant path length (500 and 100 μm for H 2 O and FFA, respectively). This procedure also allows one to rebuild cells without performing a recalibration of the method. Once the autosampler tray is loaded with samples in capped vials, the whole tray is shaken vigorously to extract the constituent of interest from the oil into the solvent, and then the samples are left to stand until phase separation occurs (∼10 min). Automated analysis of the samples is then initiated. For each successive sample in the tray, the autosampler needle punches through the Mylar liner of the cap into the upper solvent layer, which is then aspirated into the IR cell and its spectrum recorded. A “cell-full” check is performed on the spectrum by measuring the height of a suitable solvent band in a spectral region in which the component of interest does not absorb (generally, the same band as used for the path length calculation); if this height is not within specifications, the analysis is paused, and the operator is notified to check for air bubbles in the cell or incomplete cell loading. When the “cell-full” check passes, the value of the parameter of interest is automatically calculated from the calibration equation defined for the method. Figures 5 and 6 illustrate the differential spectra (solvent subtracted out) obtained for moisture and FFA standards, respectively, after extraction with the appropriate solvent. The calibration equations developed using these spectra were as follows: (3) H 2 O ( ppm ) = 3418.70 × A 3629 cm − 1 − 61.89 R 2 = 0.998 SD = 23.8 (4) FFA ( % w/w ) = 4.51 × A 1573 cm − 1 − 0.006 R 2 = 0.999 SD = 0.0010 These calibration equations were obtained using calibration standards prepared by spiking oils with the constituent of interest and extracting them with the appropriate solvent in accordance with the analytical protocol used for sample analysis. However, because the spectral analysis is performed on the upper solvent layer containing the extracted constituent, the matrix effects associated with the oils are effectively eliminated, and hence the calibration procedure could be simplified substantially by spiking the constituents directly into their respective solvents. System Evaluation and Validation In optimizing the system parameters, the crucial variable is the pump time, the objective being to minimize sample turnaround time while avoiding sample carryover. The optimum pump time is largely a function of solvent viscosity and the wash volume required to flush out the previous sample. Preliminary trials for setting the pump time simply involved the sequential analysis of low and high FFA or moisture standards. Based on this screening work, it was found that consistent results were obtained using pump times on the order of 30 s (corresponding to a sample volume of ∼15 ml), with negligible carryover (<0.5%). Subsequent to these trials, a series of three replicate sets of six oils (olive, sunflower, canola, sesame, grape seed, and safflower) of unknown FFA and moisture content were prepared for analysis. These 18 samples were loaded into the autosampler tray in random order for analysis by each method. Table 1 presents the analytical data obtained for these runs. The excellent SD values confirm that sample carryover was negligible. Subsequently, a series of validation samples were prepared by gravimetrically spiking refined olive, corn, peanut, and sunflower oils with random, but known amounts of water (100–1000 ppm) and FFA (0.1–1%). These samples were analyzed in duplicate using the appropriate FTIR method for each constituent. The FTIR data are plotted against the amounts of moisture or FFA added to these oils in Figures 7 and 8 , respectively, and the corresponding zero-regression equations (forced through the origin) are presented in Eqs. (5) and (6) (5) FTIR H 2 O = 1.007 H 2 O R 2 = 0.997 SD = 14 ppm (6) FTIR FFA = 1.011 FFA R 2 = 0.999 SD = 0.007 % FFA In a final blind validation study, 50 samples that had been pre-analyzed for FFA content in the QC department of an oil processing facility by titrimetric analysis were analyzed in our laboratory by the FTIR FFA method. The equation obtained by linear regression of the FTIR against the titrimetric data was (7) FTIR FFA = 0.011 + 1.0367 FFA R 2 = 0.978 SD = 0.015 % FFA This relationship clearly demonstrates that the FTIR method is a valid alternative to conventional titrimetric procedures. Conclusion This study provides evidence that the automated, high-speed analysis of edible oils for FFA and moisture using an FTIR spectrometer coupled to an autosampler is workable. Following sample preparation, which involves a simple in-vial extraction of the constituent in question into a low-viscosity solvent, and loading of samples onto an autosampler tray, the analysis is fully automated, with the FFA or moisture content results being output on-screen or to an LIMS. When optimized, throughputs of ∼100 samples/h can be achieved, which meet the requirements of high-volume contract, process, or payment laboratories. It is also likely that the instrument configuration described in this paper can be adapted to other FTIR-based methods such as the determination of peroxide value or trans content. Acknowledgment The authors would like to thank Sultan Qaboos University, Oman, and Thermal-Lube Inc., Pointe-Claire, PQ, Canada for their financial and equipment support, respectively, for this research. Thanks also go to Dr. R. Cocciardi for his technical assistance and Mr. Lance Shields of Casco Inc. for providing pre-analyzed corn oil samples for validation of the FFA analysis. References 1 R.D. O'Brien Fats and Oils: Formulating and Processing for Applications 1998 Technomic Publishing Company Lancaster, PA 2 AOCS Official Methods and Recommended Practices of the American Oil Chemists' Society 4th edition 1998 AOCS Press Champaign, IL 3 IUPAC Standard Methods for the Analysis of Oils, Fats and Derivatives 7th edition 1992 Blackwell Scientific London 4 A.A. Ismail F.R. van de Voort J. Sedman Rapid quantitative determination of free fatty acids in fats and oils by FTIR spectroscopy J. Am. Oil Chem. Soc. 70 1993 335 341 5 A. Al-Alawi F.R. van de Voort J. Sedman New method for the quantitative determination of free fatty acids in oil by FTIR spectroscopy J. Am. Oil Chem. Soc. 81 2004 441 446 6 H. Li F.R. van de Voort J. Sedman A.A. Ismail Rapid determination of cis and trans content, iodine value, and saponification number of edible oils by Fourier transform near-infrared spectroscopy J. Am. Oil Chem. Soc. 76 1999 491 497 7 F.R. van de Voort A.A. Ismail J. Sedman A rapid, automated method for the determination of cis and trans content of fats and oils by Fourier transform infrared spectroscopy J. Am. Oil Chem. Soc. 72 1995 873 880 8 H. Li F.R. van de Voort A.A. Ismail J. Sedman R. Cox Trans determination of edible oils by Fourier transform near-infrared spectroscopy J. Am. Oil Chem. Soc. 77 2000 1061 1067 9 J. Sedman F.R. van De Voort A.A. Ismail Simultaneous determination of iodine value and trans content of fats and oils by single-bounce horizontal attenuated total reflectance Fourier transform infrared spectroscopy J. Am. Oil Chem. Soc. 77 2000 399 403 10 J. Dubois F.R. van de Voort J. Sedman A.A. Ismail H.R. Ramaswamy Quantitative Fourier transform infrared analysis for anisidine value and aldehydes in thermally stressed oils J. Am. Oil Chem. Soc. 73 1996 787 794 11 F.R. van de Voort K.P. Memon J. Sedman A.A. Ismail Determination of solid fat index by Fourier-transform infrared spectroscopy J. Am. Oil Chem. Soc. 73 1996 411 416 12 F.R. van de Voort A.A. Ismail J. Sedman J. Dubois T. Nicodemo The determination of peroxide value by Fourier transform infrared (FTIR) spectroscopy J. Am. Oil Chem. Soc. 71 1994 921 926 13 K. Ma F.R. van de Voort J. Sedman A.A. Ismail Stoichiometric determination of hydroperoxides in fats and oils by Fourier transform infrared spectroscopy J. Am. Oil Chem. Soc. 74 1997 897 906 14 H. Li F.R. van de Voort A.A. Ismail R. Cox Determination of peroxide value by Fourier transform near-infrared spectroscopy J. Am. Oil Chem. Soc. 77 2000 137 142 15 A. Al-Alawi F.R. van de Voort J. Sedman A new FTIR method for the analysis of low levels of FFA in refined edible oils Spectrosc. Lett. 38 2005 389 403 16 A. Al-Alawi F.R. van de Voort J. Sedman A new FTIR method for the determination of low levels of moisture in edible oils Appl. Spectrosc 59 2005 1295 1299
更多查看译文
关键词
water absorption,phase separation,user interface,quantitative analysis,process control,infrared
AI 理解论文
溯源树
样例
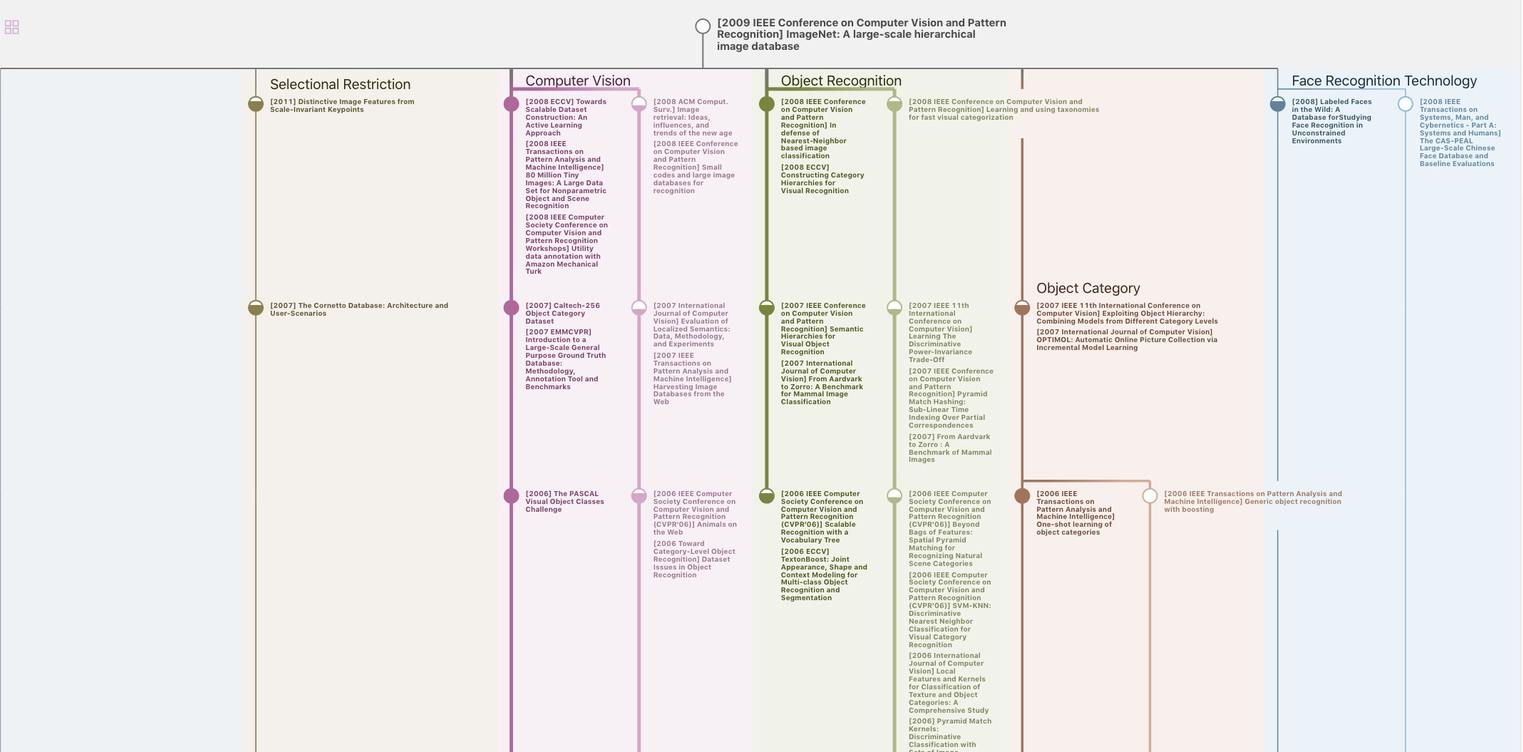
生成溯源树,研究论文发展脉络
Chat Paper
正在生成论文摘要