Role of Gap Junctions in Cardiac Conduction and Development
Circulation Research(2000)
摘要
HomeCirculation ResearchVol. 87, No. 5Role of Gap Junctions in Cardiac Conduction and Development Free AccessEditorialPDF/EPUBAboutView PDFView EPUBSections ToolsAdd to favoritesDownload citationsTrack citationsPermissions ShareShare onFacebookTwitterLinked InMendeleyReddit Jump toFree AccessEditorialPDF/EPUBRole of Gap Junctions in Cardiac Conduction and Development Insights From the Connexin Knockout Mice Cecilia W. Lo Cecilia W. LoCecilia W. Lo From the Biology Department, Goddard Laboratory, University of Pennsylvania, Philadelphia, Pa. Search for more papers by this author Originally published1 Sep 2000https://doi.org/10.1161/01.RES.87.5.346Circulation Research. 2000;87:346–348Gap junctions are membrane channels that mediate the cell-to-cell movement of ions and small metabolites. In the heart, gap junctions play an important role in impulse conduction. Studies over the last decade have revealed that gap junctions are encoded by a multigene family known as the connexins. There are at least 15 connexin genes in the vertebrate genome.1 Connexins have been referred to by 2 nomenclature systems, either according to the molecular weight of the protein or by its class as determined by protein sequence.12 The molecular weight designation will be used here in conformity with the study by Kirchhoff et al3 in this issue of Circulation Research.In the heart, there are 3 major connexin isotypes expressed: connexin (Cx)43 (α1 connexin), Cx45 (α6 connexin), and Cx40 (α5 connexin). Each of these connexins exhibits different channel properties and is regulated by different gating mechanisms. Cx43 is the only connexin known to be expressed in the adult working myocardium,4 although a recent report suggests that Cx37 (α6 connexin) also may be present.5 In the specialized conductive tissue of the heart, Cx45 is found in the atrioventricular node and adjoining His bundles.6 This connexin isotype forms voltage-sensitive channels with very low conductance.7 In contrast, Cx40, which generates channels with high conductance,8 is expressed in the fast-conducting tissues of the His-Purkinje system, being nested within the Cx45 expression domain (Figure).910 In the Purkinje fibers of the peripheral conductive tissue, Cx43 is coexpressed with Cx40 (Figure).9 It is hypothesized that this compartmentalized expression of connexins may provide for the orderly and sequential spread of activation from the atrial to ventricular chamber.91011 Thus, expression of Cx45 in the atrioventricular node and His bundle may electrically insulate the central conductive tissues from the working myocardium. In contrast, Cx40 may allow rapid impulse propagation to distal parts of the conduction system, where Cx43 may additionally facilitate coupling to the surrounding working myocytes.With the availability of the embryonic stem cell technology for targeted gene disruption, the analysis of the of role of gap junctions in cardiac conduction is now focused on the cardiac phenotype of the respective connexin knockout animal models. The first model to be made was the Cx43 knockout mouse,12 which dies neonatally from pulmonary outflow obstruction. These mice surviving to term was understandable, because 2 other connexins, Cx45 and Cx40, are expressed in the embryonic and fetal myocardium.1314 Nevertheless, the phenotype of this mouse was puzzling, because Cx43 is not known to be expressed in the outflow tract. Recent studies suggest that this cardiac phenotype may involve cardiac neural crest perturbation,1516 a migratory cell population that plays an important role in outflow tract tissue remodeling.17 The neonatal lethality of the Cx43 knockout mouse has precluded the use of electrocardiography to examine cardiac function. However, cardiac function in the viable heterozygous Cx43 knockout adult animals was examined by electrocardiography and optical mapping of surface conduction velocities. Such studies revealed no significant change in conduction velocity, activation pattern, or any other cardiac conduction parameters.18 These results were confirmed in the study by Kirchhoff et al.3 Nevertheless, slowing in the spread of activation was reported in an earlier study of the heterozygous Cx43 knockout mouse.19 An open question is whether a 50% reduction in Cx43 protein would be expected to have a significant impact on conduction velocity. Surprisingly, recent modeling studies by Jongsma and Wilders20 suggest that the answer is perhaps not to the degree one might expect. Their computer simulations indicated that even a 40% reduction in gap junction content had only modest effects on conduction velocity. Instead, cell geometry and cytoplasmic resistivity are likely to have greater roles in determining cardiac conduction velocity.21More recently, a Cx40 knockout mouse model was generated by 2 independent groups,2223 and although these mice are homozygous viable, they show slowed conduction and a partial atrioventricular block.2122 Arrhythmia was also observed, but this was found by only 1 of the 2 groups.2122 This discrepancy was attributed to strain background differences,2122 a reasonable possibility given that the 2 studies reported QTmax intervals for the wild-type littermates that were markedly different. In the study by Kirchhoff et al,3 a more detailed characterization of the Cx40 knockout mouse model is described. Also presented is a characterization of the cardiac phenotype of the Cx43/Cx40 double-knockout mice. The main conclusion reported is that Cx43 and Cx40 have additive effects on ventricular conduction and heart morphogenesis. Ventricular conduction was examined by electrocardiography, which revealed a very small increase in QRS and QTmax intervals in the double-heterozygous knockout mice (Cx43+/−/Cx40+/−) as compared with wild-type (Cx43+/+/Cx40+/+) or single-heterozygous knockout animals (Cx43+/−/Cx40+/+; Cx43+/+/Cx40+/−). These changes were proposed to arise from the combined effects of slowed conduction in the central conduction system (where Cx40 is expressed) and in the working myocytes (where Cx43 is expressed).Surprisingly, the present study also reported that some of the homozygous Cx40 knockout animals died prenatally or neonatally. Thus, half of the homozygous Cx40 knockout mice apparently died between embryonic days 12.5 and 13.5, and a few also succumbed shortly after birth or as young adults. Histological analysis of embryonic day 12.5 hearts revealed no obvious cardiac defects except for incomplete formation of the mesenchymal cap of the atrial septum. The authors suggest that prenatal lethality is likely attributable to functional, not structural, deficits in the heart. However, because Cx40 is expressed in many other tissues besides the heart, including the vasculature, the cause of prenatal lethality in the Cx40-deficient mice remains an open question. Their morphological analysis of a few newborn and adult homozygous Cx40 knockout mice revealed more substantive cardiac abnormalities, including myocardial hypertrophy, common atrioventricular junction, or, in one case, ventricular septal defects. When the Cx40 and Cx43 knockout mice were intercrossed, surprisingly, no Cx40−/−/Cx43+/− mice were obtained. Such mice apparently died neonatally. These animals exhibited cardiac septation defects similar to those seen in the homozygous Cx40 knockout mice but more severe. However, in contrast to Cx43 haploinsufficiency, which worsened the Cx40 knockout heart phenotype, Cx40+/−/Cx43−/− littermates were reported to have the same cardiac defects typically seen in the Cx40+/+/Cx43−/− animals, which consisted of malformations involving the conotruncus.12These observations led the authors to conclude that Cx43 and Cx40 have additive effects on heart septation. How this might be generated is unclear, because presently there is no known role for Cx43 or Cx40 in heart septation. Also puzzling is that the additive effects of Cx43/Cx40 deficiencies on heart septation are nonreciprocal. Perhaps the septation defects may represent secondary effects elicited by Cx43/Cx40 deficiency in the fetal myocardium or conduction system. Given that strain background effects had previously been implicated in modifying the cardiac phenotype of the Cx40 knockout mouse,3 is it possible that this may underlie the increased severity of heart septation defects in offspring obtained from intercrossing the Cx40 and Cx43 knockout mice? Ideally, this question can be resolved unambiguously by repeating the same experiment using Cx43 and Cx40 knockout mice maintained in an identical inbred strain background. However, in reality this often is not easy to orchestrate. Short of this, some insights may come from examining Cx40−/−/Cx43+/+ littermates obtained from intercrosses of the Cx43/Cx40 double-knockout mice and confirming that these mice exhibit the same mild heart-septation defects found in Cx40-deficient mice derived from the single Cx40 knockout mouse matings. Yet another experimental strategy is to interbreed Cx40+/−/Cx43+/− knockout mice (representing F1 animals) generated from the Cx40/Cx43 double-knockout intercrosses and examine the resulting F2 progeny for cardiac defects. These F2 animals should be more similar to each other in strain background than the F1 animals obtained in the initial Cx43/Cx40 knockout intercross. Resolution of this strain background issue is of tantamount importance, because resting on this is our understanding of the essential roles of connexins in cardiac development and function.The tripartite circle is now complete with the most recent production of a Cx45 knockout mouse model.24 These mice die from heart failure at embryonic day 10, exhibiting a cardiac phenotype more complex than expected. In the absence of Cx45, heart contractions were initiated. However, there was conduction block through the atrioventricular canal, and contractions in the outflow tract were not coordinated with those of the ventricle. These results are consistent with the previous studies showing that Cx45 is uniquely expressed in the atrioventricular canal and outflow tract.1325 In addition, the Cx45 knockout embryos exhibited an endocardial cushion defect, which is hypothesized to arise from a failure of cells in the endocardium to undergo the requisite epithelial-mesenchymal transformation. These studies show that Cx45 has an indispensable role in cardiac conduction during very early stages of heart development. In addition, Cx45 has a previously unrecognized role in development of the endocardial cushion. Because cardiac contractions are initiated in the absence of Cx45, these results suggest that there is yet another connexin expressed in the early heart tube.With the availability of knockout mouse models encompassing all 3 major connexins expressed in the heart, it will not be long before we learn what is the cardiac phenotype of the double- and triple-compound knockout animals. If we are to unravel the unique functions of the different connexins in heart conduction and development, we must take into account issues relating to the modification of cardiac phenotype by strain background effects. Given that these 3 connexins are expressed in many other tissues besides the heart, it is likely that altering the dosage of these 3 connexin genes simultaneously may result in early embryonic lethality, a problem that perhaps can be addressed using the Cre/loxP method for generating conditional knockout animals. A potential problem with Cre recombination is that, invariably, mosaicisms arise because of the <100% efficiency of Cre recombination. This will no doubt complicate the interpretation of conditional knockout experiments, especially those involving connexins, because connexins function in a noncell autonomous manner.Overall, these knockout mouse models have confirmed that gap junctions have important roles in cardiac conduction and heart morphogenesis. As with many other genes, the phenotypes of the connexin knockout animals have not made transparent their roles in cardiac development and function. This problem is exacerbated by the fact that most cells express multiple connexins. A central question that remains is the role of Cx43 in heart conduction. This question now can be addressed using optical mapping methods to monitor surface conduction velocities in the embryonic and fetal Cx43 knockout mouse hearts (D. Vaidya, G. Morley, and J. Jalife, personal communication, August 2000). Another outstanding question is the role of Cx45 in the central conduction system, which forms relatively late in fetal development, after the time when the Cx45 knockout mouse expires. Perhaps this developmental block can be bypassed using the Cre/loxP method of conditional gene knockout. The major challenge in the future is to relate the cardiac phenotypes of the connexin knockout animals to events occurring at the cellular and molecular levels. Only then will we begin to unravel the multiple roles of gap junctions in cardiac conduction and heart morphogenesis. The beginning of the end is here, and yet it seems like we have hardly begun.The opinions expressed in this editorial are not necessarily those of the editors or of the American Heart Association.Download figureDownload PowerPoint Figure 1. Cx43 (α1 connexin) and other connexins in the atrioventricular conduction system of the adult mouse heart. Although Cx43 is absent or coexpressed with other connexins Cx40 (α5) and Cx45 (α6), in the atria and different parts of the conduction system, including the atrioventricular node, His bundle, and Purkinje fibers, Cx43 is the only connexin expressed at significant levels by the working ventricular myocardium. Note that cellular domains of connexin expression along the atrioventricular conduction axis are depicted as distinct segment-like compartments. Reprinted with permission from Gourdie and Lo.6My laboratory is generously supported by grants from the National Science Foundation, National Institute of Child Health and Human Development, and National Heart, Lung, and Blood Institute. I am grateful to Maja Bucan and Robert Gourdie for helpful discussions on issues relating to the genetics of the knockout mouse crosses and the role of connexins in cardiac conduction, respectively.FootnotesCorrespondence to Cecilia W. Lo, Biology Department, Goddard Laboratory, University of Pennsylvania, Philadelphia, PA 19104-6017. E-mail [email protected] References 1 Bruzzone R, White TW, Paul DL. Connections with connexins: the molecular basis of direct intercellular signaling. Eur J Biochem.1996; 238:1–27.CrossrefMedlineGoogle Scholar2 Kumar NM, Gilula NB. The gap junction communication channel. Cell.1996; 84:381–388.CrossrefMedlineGoogle Scholar3 Kirchhoff S, Kim J-S, Hagendorff A, Thönnissen E, Krueger O, Lamers WH, Willecke K. Abnormal cardiac conduction and morphogenesis in connexin40 and connexin43 double-deficient mice. Circ Res.2000; 87:399–405.CrossrefMedlineGoogle Scholar4 Van Kempen MJ, Fromaget C, Gros D, Moorman AF, Lamers WH. Spatial distribution of connexin43, the major cardiac gap junction protein, in the developing and adult rat heart. Circ Res.1991; 68:1638–1651.CrossrefMedlineGoogle Scholar5 Haefliger JA, Polikar R, Schnyder G, Burdet M, Sutter E, Pexieder T, Nicod P, Meda P. Connexin37 in normal and pathological development of mouse heart and great arteries. Dev Dyn.2000; 218:331–344.CrossrefMedlineGoogle Scholar6 Gourdie RG, Lo CW. Cx43 (α1) gap junctions in cardiac development and disease. Curr Top Membr.2000; 49:581–602.Google Scholar7 Veenstra RD, Wang HZ, Westphale EM, Beyer EC. Multiple connexins confer distinct regulatory and conductance properties of gap junctions in developing heart. Circ Res..1992; 71:1277–1283.CrossrefMedlineGoogle Scholar8 Beblo DA, Veenstra RD. Monovalent cation permeation through the connexin40 gap junctional channel. J Gen Physiol.1997; 109:509–522.CrossrefMedlineGoogle Scholar9 Gourdie RG, Severs NJ, Green CR, Rothery S, Germroth P, Thompson RP. The spatial distribution and relative abundance of gap junctional connexin40 and connexin43 correlate to functional properties of components of the cardiac atrioventricular conduction system. J Cell Sci.1993; 105:985–991.CrossrefMedlineGoogle Scholar10 Coppen SR, Severs NJ, Gourdie RG. Connexin45 delineates an extended conduction system in the embryonic and mature rodent heart. Dev Genet.1999; 24:82–90.CrossrefMedlineGoogle Scholar11 Gros DB, Jongsma HJ. Connexins in mammalian heart function. Bioessays.1996; 18:719–730.CrossrefMedlineGoogle Scholar12 Reaume AG, de Sousa PA, Kulkarni S, Langille BL, Zhu D, Davies TC, Juneja SC, Kidder GM, Rossant J. Cardiac malformation in neonatal mice lacking connexin43. Science.1995; 267:1831–1834.CrossrefMedlineGoogle Scholar13 Delorme B, Dahl E, Jarry-Guichard T, Briand JP, Willecke K, Gros D, Theveniau-Ruissy M. Expression pattern of connexin gene products at the early developmental stages of the mouse cardiovascular system. Circ Res.1997; 81:423–437.CrossrefMedlineGoogle Scholar14 Van Kempen MJ, Mermeulen JL, Moorman AFM, Gros D, Paul DL, Lamers WH. Developmental changes of connexin40 and connexin43 mRNA distribution patterns in the rat heart. Cardiovasc Res.1996; 32:886–900.CrossrefMedlineGoogle Scholar15 Ewart JL, Cohen MF, Wessels A, Gourdie RG, Chin AJ, Park SMJ, Lazatin BO, Villabon S, Lo CW. Heart and neural tube defects in transgenic mice overexpressing the Cx43 gap junction gene. Development.1997; 124:1281–1292.CrossrefMedlineGoogle Scholar16 Huang GY, Cooper ES, Waldo K, Kirby ML, Gilula NB, Lo CW. Gap junction mediated cell-cell communication modulates mouse neural crest migration. J Cell Biol.1998; 143:1725–1734.CrossrefMedlineGoogle Scholar17 Kirby ML, Waldo KL. Neural crest and cardiovascular patterning. Circ Res.1995; 77:211–215.CrossrefMedlineGoogle Scholar18 Morley GE, Vaidya D, Samie FH, Lo CW, Delmar M, Jalife J. Characterization of conduction in the ventricles of normal and heterozygous Cx43 knockout mice using optical mapping. J Cardiovasc Electrophysiol.1999; 10:1361–1375.CrossrefMedlineGoogle Scholar19 Guerrero PA, Schuessler RB, Davis LM, Beyer EC, Johnson CM, Yamada KA, Saffitz JE. Slow ventricular conduction in mice heterozygous for a connexin43 null mutation. J Clin Invest.1997; 99:1991–1998.CrossrefMedlineGoogle Scholar20 Jongsma HJ, Wilders R. Gap junctions in cardiovascular disease. Circ Res.2000; 86:1193–1197.CrossrefMedlineGoogle Scholar21 Spach MS, Heidlage JF, Dolber PC, Barr RC. Electrophysiological effects of remodeling cardiac gap junctions and cell size: experimental and model studies of normal cardiac growth. Circ Res.2000; 86:302–311.CrossrefMedlineGoogle Scholar22 Kirchhoff S, Nelles E, Hagendorff A, Kruger O, Traub O, Willecke K. Reduced cardiac conduction velocity and predisposition to arrhythmias in connexin40-deficient mice. Curr Biol.1998; 8:229–302.Google Scholar23 Simon AM, Goodenough DA, Paul DL. Mice lacking connexin40 have cardiac conduction abnormalities characteristic of atrioventricular block and bundle branch block. Curr Biol.1998; 8:295–298.CrossrefMedlineGoogle Scholar24 Kumai M, Nishii K, Nakamura KI, Takeda N, Suzuki M, Shibata Y. Loss of connexin45 causes a cushion defect in early cardiogenesis. Development.2000; 127:3501–3512.CrossrefMedlineGoogle Scholar25 Alcolea S, Theveniau-Ruissy M, Jarry-Guichard T, Marics I, Tzouanacou E, Cauvin JP, Briand JP, Moorman AFM. Lamers WH, Gros DB. Down regulation of connexin45 gene products during mouse heart development. Circ Res.1999; 84:1364–1379.Google Scholar Previous Back to top Next FiguresReferencesRelatedDetailsCited By Park Y, Park B, Choi H, Lee S, Kim M, Park H and Kim I (2022) Chorion-derived perinatal mesenchymal stem cells improve cardiac function and vascular regeneration: Preferential treatment for ischemic heart disease, Hellenic Journal of Cardiology, 10.1016/j.hjc.2022.05.010, Online publication date: 1-May-2022. Yin Y, Zhong Y, Zhu Y and Tian L (2022) Changes in gap junction proteins Connexin30.2 and Connexin40 expression in the sinoatrial node of rats with dexmedetomidine-induced sinus bradycardia, Brazilian Journal of Anesthesiology (English Edition), 10.1016/j.bjane.2022.05.004, Online publication date: 1-May-2022. Kiss E, Fischer C, Sauter J, Sun J and Ullrich N (2022) The Structural and the Functional Aspects of Intercellular Communication in iPSC-Cardiomyocytes, International Journal of Molecular Sciences, 10.3390/ijms23084460, 23:8, (4460) Trosko J (2021) In Search of a Unifying Concept in Human Diseases, Diseases, 10.3390/diseases9040068, 9:4, (68) Guo F, Hall A, Tape C, Ling S and Pointon A (2021) Intra- and intercellular signaling pathways associated with drug-induced cardiac pathophysiology, Trends in Pharmacological Sciences, 10.1016/j.tips.2021.05.004, 42:8, (675-687), Online publication date: 1-Aug-2021. Trosko J (2021) On the potential origin and characteristics of cancer stem cells, Carcinogenesis, 10.1093/carcin/bgab042, 42:7, (905-912), Online publication date: 16-Jul-2021. Rodríguez-Sinovas A, Sánchez J, Valls-Lacalle L, Consegal M and Ferreira-González I (2021) Connexins in the Heart: Regulation, Function and Involvement in Cardiac Disease, International Journal of Molecular Sciences, 10.3390/ijms22094413, 22:9, (4413) Hendrickson T, Mancino C, Whitney L, Tsao C, Rahimi M and Taraballi F (2021) Mimicking cardiac tissue complexity through physical cues: A review on cardiac tissue engineering approaches, Nanomedicine: Nanotechnology, Biology and Medicine, 10.1016/j.nano.2021.102367, 33, (102367), Online publication date: 1-Apr-2021. Saadeh K and Fazmin I (2021) Mitochondrial Dysfunction Increases Arrhythmic Triggers and Substrates; Potential Anti-arrhythmic Pharmacological Targets, Frontiers in Cardiovascular Medicine, 10.3389/fcvm.2021.646932, 8 Bhattacharyya S and Munshi N (2020) Development of the Cardiac Conduction System, Cold Spring Harbor Perspectives in Biology, 10.1101/cshperspect.a037408, 12:12, (a037408), Online publication date: 1-Dec-2020. Xiao S, Shimura D, Baum R, Hernandez D, Agvanian S, Nagaoka Y, Katsumata M, Lampe P, Kleber A, Hong T and Shaw R (2020) Auxiliary trafficking subunit GJA1-20k protects connexin-43 from degradation and limits ventricular arrhythmias, Journal of Clinical Investigation, 10.1172/JCI134682, 130:9, (4858-4870), Online publication date: 10-Aug-2020., Online publication date: 1-Sep-2020. Bousalis D, Lacko C, Hlavac N, Alkassis F, Wachs R, Mobini S, Schmidt C and Kasahara H (2020) Extracellular Matrix Disparities in an Nkx2-5 Mutant Mouse Model of Congenital Heart Disease, Frontiers in Cardiovascular Medicine, 10.3389/fcvm.2020.00093, 7 Zlabinger K, Spannbauer A, Traxler D, Gugerell A, Lukovic D, Winkler J, Mester-Tonczar J, Podesser B and Gyöngyösi M (2019) MiR-21, MiR-29a, GATA4, and MEF2c Expression Changes in Endothelin-1 and Angiotensin II Cardiac Hypertrophy Stimulated Isl-1+Sca-1+c-kit+ Porcine Cardiac Progenitor Cells In Vitro, Cells, 10.3390/cells8111416, 8:11, (1416) Zhang X, Chen D, Wang J, Liu J, Guo H and Zhang G (2019) Involvement of sphingosine-1-phosphate receptors 2/3 in IR-induced sudden cardiac death, Heart and Vessels, 10.1007/s00380-018-01323-8, 34:6, (1052-1063), Online publication date: 1-Jun-2019. Dilaveris P, Antoniou C, Manolakou P, Tsiamis E, Gatzoulis K and Tousoulis D Biomarkers Associated with Atrial Fibrosis and Remodeling, Current Medicinal Chemistry, 10.2174/0929867324666170918122502, 26:5, (780-802) Lluri G and Deb A (2019) WNT Signaling and Cardiac Fibrosis Fibrosis in Disease, 10.1007/978-3-319-98143-7_11, (319-334), . Rommel C, Rösner S, Lother A, Barg M, Schwaderer M, Gilsbach R, Bömicke T, Schnick T, Mayer S, Doll S, Hesse M, Kretz O, Stiller B, Neumann F, Mann M, Krane M, Fleischmann B, Ravens U and Hein L (2018) The Transcription Factor ETV1 Induces Atrial Remodeling and Arrhythmia, Circulation Research, 123:5, (550-563), Online publication date: 17-Aug-2018. Ock S, Lee W, Kim H, Park K, Kim Y, Kook H, Park W, Lee T, Abel E and Kim J (2018) Connexin43 and zonula occludens-1 are targets of Akt in cardiomyocytes that correlate with cardiac contractile dysfunction in Akt deficient hearts, Biochimica et Biophysica Acta (BBA) - Molecular Basis of Disease, 10.1016/j.bbadis.2018.01.022, 1864:4, (1183-1191), Online publication date: 1-Apr-2018. Johnson R and Camelliti P (2018) Role of Non-Myocyte Gap Junctions and Connexin Hemichannels in Cardiovascular Health and Disease: Novel Therapeutic Targets?, International Journal of Molecular Sciences, 10.3390/ijms19030866, 19:3, (866) Foulquier S, Daskalopoulos E, Lluri G, Hermans K, Deb A, Blankesteijn W and Michel M (2017) WNT Signaling in Cardiac and Vascular Disease, Pharmacological Reviews, 10.1124/pr.117.013896, 70:1, (68-141), Online publication date: 1-Jan-2018. Anjo S, Martins-Marques T, Pereira P, Girão H and Manadas B (2018) Elucidation of the dynamic nature of interactome networks: A practical tutorial, Journal of Proteomics, 10.1016/j.jprot.2017.04.011, 171, (116-126), Online publication date: 1-Jan-2018. Kahane A, Park A and Ray J (2018) Dysfunctional Uterine Activity in Labour and Premature Adverse Cardiac Events: Population-Based Cohort Study, Canadian Journal of Cardiology, 10.1016/j.cjca.2017.10.007, 34:1, (45-51), Online publication date: 1-Jan-2018. Epifantseva I and Shaw R (2018) Intracellular trafficking pathways of Cx43 gap junction channels, Biochimica et Biophysica Acta (BBA) - Biomembranes, 10.1016/j.bbamem.2017.05.018, 1860:1, (40-47), Online publication date: 1-Jan-2018. Shaw R (2018) Ion Channel Trafficking in the Heart Cardiac Electrophysiology: From Cell to Bedside, 10.1016/B978-0-323-44733-1.00017-1, (160-166), . Tirziu D (2018) Cardiac Hypertrophy: Signaling and Cellular Crosstalk Encyclopedia of Cardiovascular Research and Medicine, 10.1016/B978-0-12-809657-4.99834-X, (434-450), . Tirziu D (2018) OBSOLETE: Cardiac Hypertrophy: Signaling and Cellular Crosstalk Reference Module in Biomedical Sciences, 10.1016/B978-0-12-801238-3.99834-9, . Dabbagh A, Imani A and Rajaei S (2018) Cardiac Physiology Postoperative Critical Care for Adult Cardiac Surgical Patients, 10.1007/978-3-319-75747-6_3, (25-74), . Lee H, Chen C, Tsai W, Lin H, Shiao Y, Sheu S, Wu B, Chen C and Lai W (2017) Very-Low-Density Lipoprotein of Metabolic Syndrome Modulates Gap Junctions and Slows Cardiac Conduction, Scientific Reports, 10.1038/s41598-017-11416-5, 7:1, Online publication date: 1-Dec-2017. Lee C, Choi J, Shin S, Lee J, Seo H, Lim S, Lee S, Joo H, Kim S and Hwang K (2017) Interaction of small G protein signaling modulator 3 with connexin 43 contributes to myocardial infarction in rat hearts, Biochemical and Biophysical Research Communications, 10.1016/j.bbrc.2017.07.081, 491:2, (429-435), Online publication date: 1-Sep-2017. Calkins T and Piermarini P (2017) Mosquito Gap Junctions: Molecular Biology, Physiology, and Potential for Insecticide Development Advances in Agrochemicals: Ion Channels and G Protein-Coupled Receptors (GPCRs) as Targets for Pest Control, 10.1021/bk-2017-1264.ch006, (91-110), Online publication date: 1-Jan-2017. Ongstad E and Gourdie R (2016) Can heart function lost to disease be regenerated by therapeutic targeting of cardiac scar tissue?, Seminars in Cell & Developmental Biology, 10.1016/j.semcdb.2016.05.020, 58, (41-54), Online publication date: 1-Oct-2016. Basheer W and Shaw R (2016) The “tail” of Connexin43: An unexpected journey from alternative translation to trafficking, Biochimica et Biophysica Acta (BBA) - Molecular Cell Research, 10.1016/j.bbamcr.2015.10.015, 1863:7, (1848-1856), Online publication date: 1-Jul-2016. Diezmos E, Bertrand P and Liu L (2016) Purinergic Signaling in Gut Inflammation: The Role of Connexins and Pannexins, Frontiers in Neuroscience, 10.3389/fnins.2016.00311, 10 Basheer W and Shaw R (2016) Connexin 43 and CaV1.2 Ion Channel Trafficking in Healthy and Diseased Myocardium, Circulation: Arrhythmia and Electrophysiology, 9:6, (e001357), Online publication date: 1-Jun-2016. Martins-Marques T, Anjo S, Pereira P, Manadas B and Girão H (2015) Interacting Network of the Gap Junction (GJ) Protein Connexin43 (Cx43) is Modulated by Ischemia and Reperfusion in the Heart*, Molecular & Cellular Proteomics, 10.1074/mcp.M115.052894, 14:11, (3040-3055), Online publication date: 1-Nov-2015. Hara M, Takahashi T, Mitsumasu C, Igata S, Takano M, Minami T, Yasukawa H, Okayama S, Nakamura K, Okabe Y, Tanaka E, Takemura G, Kosai K, Yamashita Y and Matsuishi T (2015) Disturbance of cardiac gene expression and cardiomyocyte structure predisposes Mecp2-null mice to arrhythmias, Scientific Reports, 10.1038/srep11204, 5:1, Online publication date: 1-Sep-2015. Orenes-Piñero E, Quintana-Giner M, Romero-Aniorte A, Valdés M and Marín F (2015) Novel biomarkers in cardiology: MicroRNAs in atrial fibrillation, Archivos de Cardiología de México, 10.1016/j.acmx.2015.01.005, 85:3, (225-229), Online publication date: 1-Jul-2015. Hermans K and Blankesteijn W (2015) Wnt Signaling in Cardiac Disease Comprehensive Physiology, 10.1002/cphy.c140060, (1183-1209) Yang K, Kyle J, Makielski J and Dudley S (2015) Mechanisms of Sudden Cardiac Death, Circulation Research, 116:12, (1937-1955), Online publication date: 5-Jun-2015.Fry C, Gray R, Dhillon P, Jabr R, Dupont E, Patel P and Peters N (2014) Architectural Correlates of Myocardial Conduction, Circulation: Arrhythmia and Electrophysiology, 7:6, (1198-1204), Online publication date: 1-Dec-2014. Yang K, Bonini M and Dudley S (2014) Mitochondria and arrhythmias, Free Radical Biology and Medicine, 10.1016/j.freeradbiomed.2014.03.033, 71, (351-361), Online publication date: 1-Jun-2014. Martins A, Eng G, Caridade S, Mano J, Reis R and Vunjak-Novakovic G (2014) Electrically Conductive Chitosan/Carbon Scaffolds for Cardiac Tissue Engi
更多查看译文
AI 理解论文
溯源树
样例
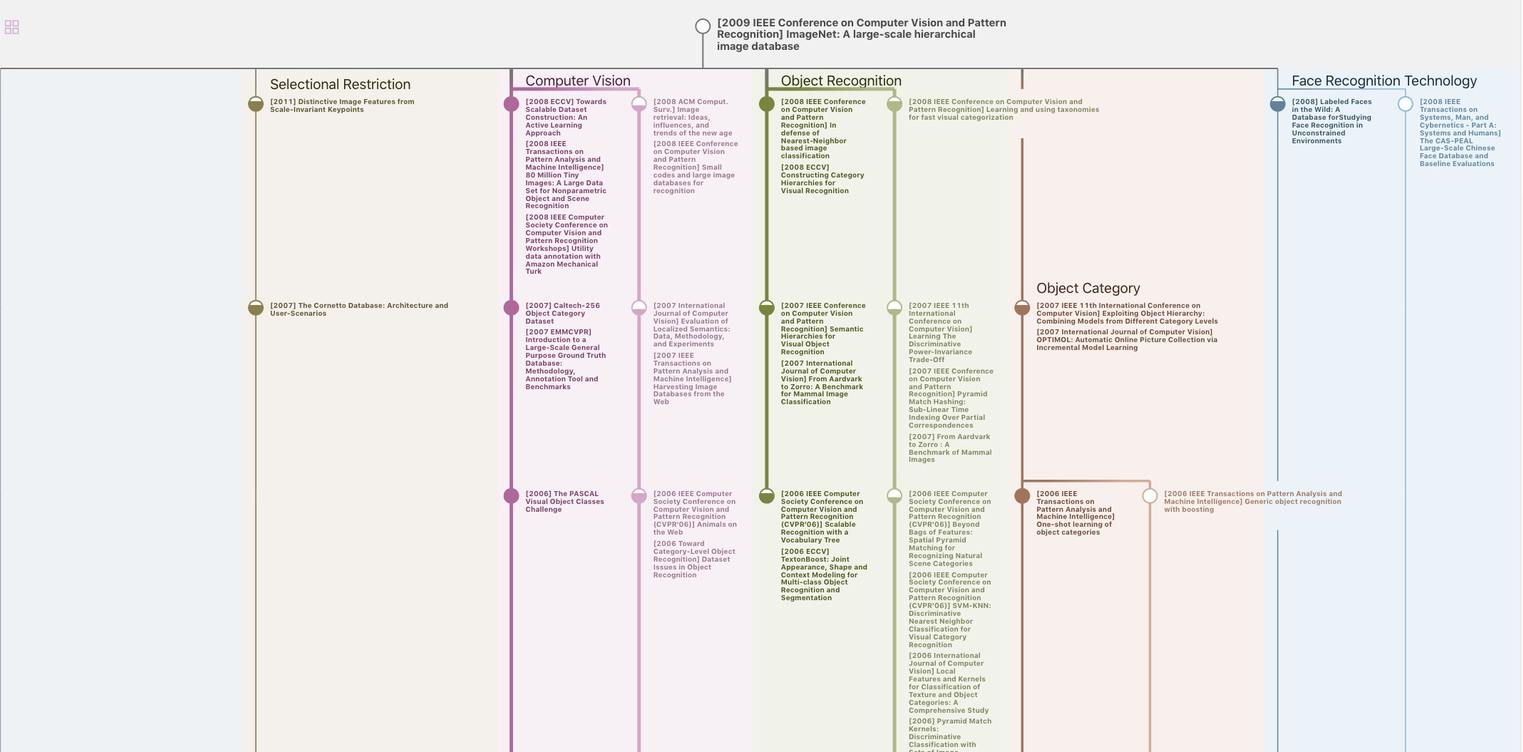
生成溯源树,研究论文发展脉络
Chat Paper
正在生成论文摘要