Feasibility of a Colliding Beam Fusion Reactor
science(2010)
摘要
N. Rostoker, M. W. Binderbauer, and H. J. Monkhorst (1) revisit several ideas—the use of the reactions of protons with boron-11 (p-B) to produce fusion energy, (2) the use of the field-reversed configuration (FRC) for magnetic confinement of particles and energy (E) (3), and the use of nonthermal ion distributions to enhance the fusion reactivity (4)— in their proposal for a colliding beam fusion reactor (CBFR). While there are unresolved issues with each of these choices, I focus here on nonthermal ion distributions. The fundamental difficulty with nonthermal ions is apparent when one compares the fusion cross section (sfusion ' 1 barn for p-B at Ecm 5 580 keV, where Ecm is the energy in the center-of-mass frame) to the effective cross section for many small-angle Coulomb scattering events that combine to produce a scattering angle of 90° rms in an incident beam (seff ' 60 barns for protons scattering on B at Ecm 5 580 keV). Highly nonthermal systems, like the colliding beam reactor proposed by Rostoker et al. would relax to local thermal equilibrium before a significant amount of fusion power could be produced. Alternatively, the nonthermal ion distribution could be maintained by cycling sufficient power through the system. With this possibility in mind, it is instructive to examine the operating point presented by Rostoker et al. (np 5 4 3 10 /cm, nB 5 1 3 10/cm, Tp 5 25 keV, and Te 5 20 keV), where np is the proton density, nB is the boron density, Tp is the temperature of the protons, and Te is the temperature of the electrons. We consider the problem in the frame-of-reference of the protons. Maintaining Ecm 5 580 keV would require that the boron beam velocity be uB ' 1.1 3 10 9 cm/s. Coulomb collisions with the boron beam would heat the proton distribution at a rate (5) of 1/2mBuB nBy« B/p ' 500 W/cm, where mB 5 11 AMU is the boron mass and y« i/j is the energy transfer rate from collisions between particles of species “i” and “j.” As Rostoker et al. suggest, heat would be removed from the protons by collisions with the (colder) electrons at a rate of 3/2np(Tp 2 Tc) y« p/c ' 350 W/cm. The relatively small net heating of the proton distribution could be eliminated if Te were reduced to 18.6 keV. The largest term in the electron power balance is direct heating by the boron beam, which would proceed at the rate of 1/2mBuB nBy« B/e ' 2.0 kW/cm. Maintaining the electrons at Te ' 18.6 keV would require that Pelectron ' 2.5 kW/cm 3 be extracted from the electron distribution. While bremsstrahlung could provide only 44 W/cm of this electron cooling, experience suggests that it would not be difficult to find other channels for electron heat loss. The 2.5 kW/ cm extracted from the electrons must be balanced by ion heating. The fusion power (Pfusion) calculated by Rostoker et al. at the operating point is Pfusion 5 npnBYp-11B^sp-11Bv& ' 45 W/cm , where Yp-11B 5 8.7 MeV is the energy yield per fusion event (and v is the velocity of the proton and boron nuclei). The difference between Pelectron and Pfusion sets a lower limit on the external ion heating power. Thus, the fusion gain (which is normally defined as the ratio of the fusion power to the external heating power), Q [ Pfusion/Pheat # Pfusion/ (Pelectron 2 Pfusion) ' 0.02 would be much lower than the value of 2.7 stated by Rostoker et al. (1). Highly nonthermal fusion schemes generally suffer from an unattractive power balance (4), and so magnetic fusion research has focused on systems that are in local thermal equilibrium. In such systems, high fusion gain (Q .. 1) remains a distinct possibility. W. M. Nevins Lawrence Livermore National Laboratory, 7000 East Avenue, Livermore, CA 94550, USA
更多查看译文
AI 理解论文
溯源树
样例
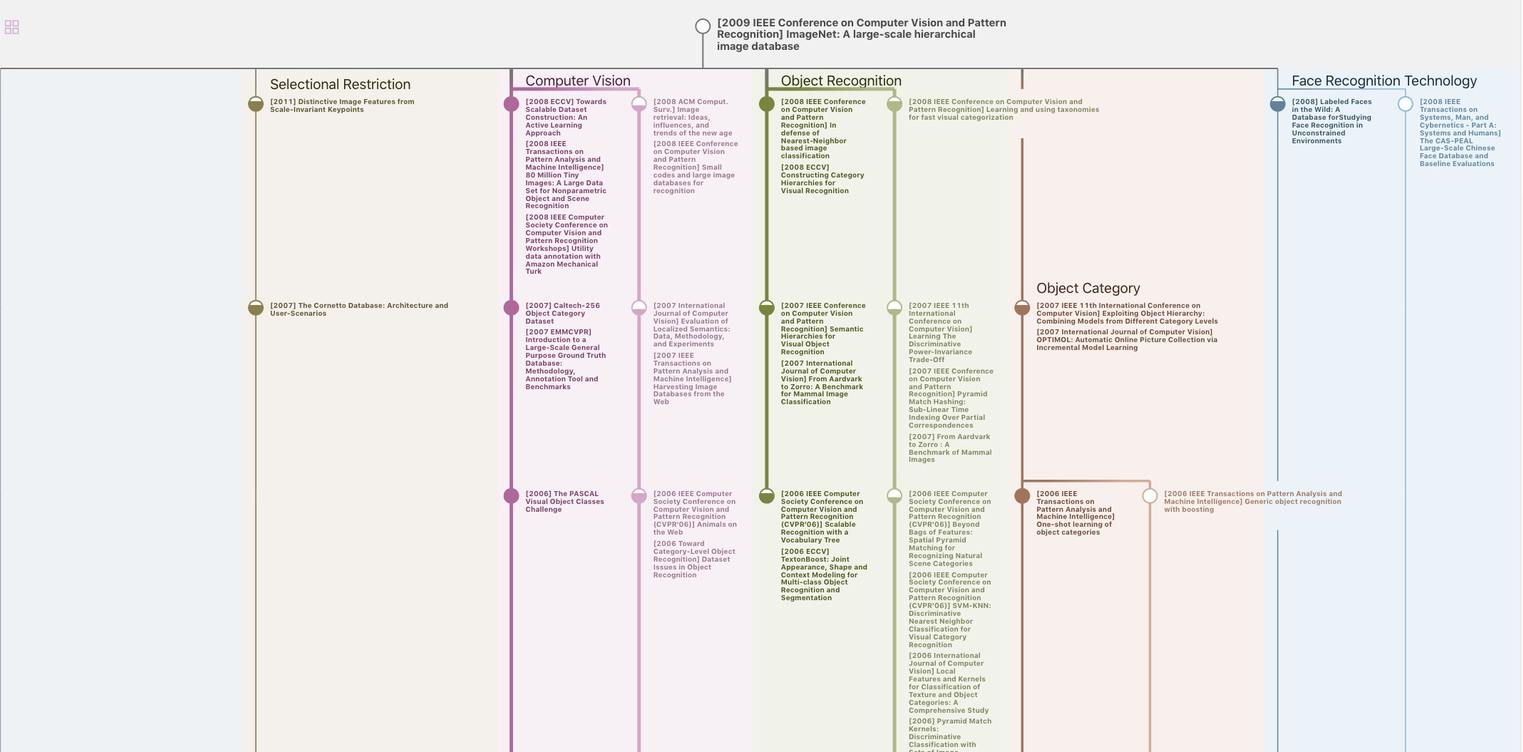
生成溯源树,研究论文发展脉络
Chat Paper
正在生成论文摘要