Polarized spectroscopic properties of a potential self-frequency doubling crystal, Nd3+:BaCaBO3F
Optical Materials(2011)
摘要
In this paper, the polarized absorption and fluorescence spectra of Nd 3+ ion in the BaCaBO 3 F non-linear optical crystal have been investigated at room temperature. The Judd–Ofelt theory, extended to anisotropic system, has been applied to calculate the phenomenological intensity parameters, spontaneous transition probabilities, branching ratios and radiative lifetimes. The maximum emission cross-sections have been calculated to be 7.22 × 10 −20 cm 2 and 15.21 × 10 −20 cm 2 at 1068 nm for π - and σ -polarization using the Füchtbauer–Ladenburg (F–L) equation. The fluorescence lifetime of 4 F 3/2 manifold is equal to 57.7 μs, and the quantum efficiency is 26.8%, which is higher than those of most of other borates. The potential of Nd 3+ :BaCaBO 3 F as a new self-frequency doubling laser crystal has also been evaluated preliminarily. Appreciable self-absorption at the second-harmonic wavelength of Nd 3+ :BaCaBO 3 F crystal should be considered for further laser operations. Keywords Solid-state laser materials Fluoborate Optical microscopy Frequency doubling 1 Introduction The present demand for small compact visible lasers has led to an increasing interest in laser diode pumping solid-state lasers based on self-frequency doubling (SFD) crystals. In SFD material, the laser effect and the non-linear optical phenomena occur simultaneously inside the same host, resulting in simple, compact and robust devices. The first demonstration of SFD laser action in the visible was from Nd:MgO:LiNbO 3 crystal in 1986 [1] . Unfortunately, the MgO codopant, which is indispensable to avoid the optical photorefractive damage, prevents the incorporation of a high Nd 3+ concentration and reduces the optical quality of the crystals. Another promising SFD crystal is Nd 3+ :YAl 3 (BO 3 ) 4 crystal due to its large non-linear coefficients, high emission cross-section, and high damage threshold [2,3] . However, it suffers from poor optical quality and self-absorption at the second-harmonic wavelength [4] . The alternatives, Nd 3+ :LnCa 4 O(BO 3 ) 3 crystals, can be grown by the Czochralski method, but the low absorption and emission cross-sections of these crystals are unfavorable for the laser conversion efficiency [5,6] . Therefore, searching for new SFD crystals is the key problem in this research field [7,8] . Early in 1994, Keszler et al. [9] reported the crystal structure of BaCaBO 3 F, where the basic structural units are the triangular (BO 3 ) 3− groups responsible for the non-linear optical effect. In 1996, Yb 3+ :BaCaBO 3 F were investigated as a new laser crystal with potential for SFD [10] . The main advantages offered by the Yb 3+ :BaCaBO 3 F crystal included the long energy storage time of Yb 3+ , the absence of absorption at the second-harmonic wavelength, and the combined second-harmonic generation and laser features available from the crystal. With a Ti:sapphire pumping source, slope efficiency up to 38% was obtained for the fundamental laser output (1034 nm). Recently, BCBF crystals with large size were grown by the Kyropoulos and Czochralski method, and the detailed linear and non-linear optical properties of this material were presented [11–13] . This paper investigates the polarized spectroscopic properties of Nd 3+ :BaCaBO 3 F crystal, and evaluates preliminarily the potential of Nd 3+ :BaCaBO 3 F as a new SFD laser crystal. 2 Experimental 2.1 Crystal growth BaCaBO 3 F melts congruently [11,13] . According, Nd 3+ :BaCaBO 3 F crystal was grown by the Czochralski method. The chemicals used were Nd 2 O 3 with purity of 99.99%, and BaCO 3 , CaCO 3 , Na 2 CO 3 , H 3 BO 3 , BaF 2 with AR grade. Owing to Nd 3+ replaced the divalent cation Ca 2+ , Na + was codoped as a charge compensator element so as to ensure the lattice electric neutrality and to increase the efficiency of dopant incorporation. In order to avoid the formation of Ba and/or Ca peroxide which attack the crucible, a two-step process was adopted for preparing a melt from which a crystal could be grown [10] , as described by the following reaction (1) BaCO 3 + 2 ( 1 - 2 x ) CaCO 3 + x Na 2 CO 3 + x Nd 2 O 3 + 2 H 3 BO 3 = BaCa 2 ( 1 - 2 x ) Na 2 x Nd 2 x ( BO 3 ) 2 + 3 H 2 O + 3 ( 1 - x ) CO 2 ↑ (2) BaCa 2 ( 1 - 2 x ) Na 2 x Nd 2 x ( BO 3 ) 2 + BaF 2 = 2 BaCa 1 - 2 x Na x Nd x BO 3 F where x = 0.02. It was noteworthy that the solid-state reaction and crystal growth were carried out in an N 2 atmosphere to prevent the formation of peroxides and the decomposition of fluorides to oxides [10] . The synthesized raw materials of Nd 3+ :BaCaBO 3 F were obtained in appropriate temperature, and then melted in a platinum crucible with dimensions of Ø 45 × 40 mm 3 . The crystal was grown in a 2 kHz frequency furnace in N 2 atmosphere. After repeating and adjusting the furnace power, Nd 3+ :BaCaBO 3 F crystal was grown at a pulling rate of 0.5 mm/h and a rotating rate of 20 rpm. When the growth process ended, the grown crystal was cooled to the room temperature at an annealing rate of 10–30 °C/h. Although the crystal was substantially fractured, single crystal of sufficient size was available for polarized spectroscopic measurements. The cracking behavior could also be found in Yb 3+ :BaCaBO 3 F crystal [10] . Considering the BaCaBO 3 F crystals with ample size and high optical quality have been grown successfully in Refs. [11–13] , we put more focus on the spectral properties rather than the crystal growth. The Nd 3+ and Na + concentrations in Nd 3+ :BaCaBO 3 F crystals were measured by the inductively coupled plasma and atomic emission spectrometry (ICP-AES, Ultima2, Jobin–Yvon). The phase of the as-grown Nd 3+ :BaCaBO 3 F crystal was checked by the X-ray powder diffraction. The XRD were performed using a X’Pert Pro powder diffractometer diffractometer and Cu Kα radiation ( λ = 1.54056 Å) at room temperature. 2.2 Spectroscopic measurements A 2.4 mm thick plate sample cut from the as-grown crystal with the c -axis contained in its main faces was applied to the spectral measurements. The crystal orientations were determined using an YX-2 type Orientation Unit. In the experiments the σ - and π -polarization were defined in terms of the E -vector being perpendicular or parallel to the c -axis, respectively. The polarized absorption spectra were recorded using a Perkin–Elmer UV–VIS-NIR Spectrometer (Lambda-900) in a range of 330–950 nm wavelengths at room temperature. The polarized fluorescence spectra and fluorescence lifetime were determined using an Edinburgh Analytical Instruments FLS920 Fluorescence Spectrophotometer with a continuous Xe-flash lamp. All the experiments were carried out at room temperature. 3 Results and discussions 3.1 Efficient segregation coefficient The Nd 3+ and Na + concentrations in Nd 3+ :BaCaBO 3 F crystals were measured to be 0.619 wt.% and 0.084 wt.%, i.e. 1.10 at.% and 0.93 at.%, respectively. The Nd 3+ ion substitutes onto an irregular seven-coordinate Ca 2+ , which is surrounded by five O 2− and two F − [13] . The small distinct between Nd 3+ and Na + concentrations indicates that the charge-compensation mechanism (Re 3+ + O 2− → Ca 2+ + F − ) happened in Yb 3+ :BaCaBO 3 F crystal may also take place in Nd 3+ :BaCaBO 3 F crystal [10] . The particular substitutional environment and the charge-compensation mechanism give rise to large crystal field splittings for the active ion and also to strongly polarized spectroscopic features, both of which turn out to be favorable for laser performance [10] . The segregation coefficient K of Nd 3+ ion in the crystal can be expressed as (3) K = C sol / C liq where C sol and C liq are the concentrations of Nd 3+ ions in the solid and liquid phases, respectively. Thus, the segregation coefficient K of Nd 3+ in BaCaBO 3 F crystal is 0.55. 3.2 XRD analysis The XRD pattern of Nd 3+ :BaCaBO 3 F crystal matches well with that of the pure BaCaBO 3 F [11] , as shown in Fig. 1 . This result confirms the grown crystal belongs to Nd 3+ :BaCaBO 3 F crystal and Nd 3+ , Na + are incorporated into the lattice. 3.3 Absorption spectra and J–O analysis Fig. 2 illustrates the polarized absorption spectra of Nd 3+ :BaCaBO 3 F crystal at room temperature. The baselines in the spectra, which are caused by the Fresnel reflection of the crystal surface and the defects in the crystal, have been corrected. All the absorption bands are attributed to the transitions of Nd 3+ ions from the ground state 4 I 9/2 to the excited state J ′ manifolds, which are marked in Fig. 2 . As can be seen, the absorption spectra show strong polarization dependence due to the anisotropy of the crystal, giving stronger line with σ -polarization. The most interesting feature in the absorption spectra is the strong absorption band around 808 nm corresponding to the 4 I 9/2 → 4 F 5/2 + 2 H 9/2 transition, which is close to the laser output of GaAlAs diode laser. The absorption peak is at 813 nm with a full width at half maximum (FWHM) of 2.9 nm for σ -polarization, which is larger than those of Nd 3+ :YVO 4 (2.0 nm) [14] and Nd 3+ :YAG (1.0 nm) crystals [15] and comparable to those of Nd 3+ :Ca 4 GdO(BO 3 ) 4 (3.0 nm) and Nd 3+ :YAl 3 (BO 3 ) 4 (3.6 nm) [16] . The broad bandwidth means that Nd 3+ :BaCaBO 3 F crystal is more suitable for diode pumping. The absorption cross-section at 813 nm for σ -polarization is 11.94 × 10 −20 cm 2 , which is not only higher than those of Nd 3+ :Ca 4 GdO(BO 3 ) 4 (2.23 × 10 −20 cm 2 for E//X) [5] , Nd 3+ :Ca 4 YO(BO 3 ) 4 (2.00 × 10 −20 cm 2 for E//Z) [6] , Nd 3+ :GdAl 3 (BO 3 ) 4 (4.30 × 10 −20 cm 2 for σ -polarization) [17] and Nd 3+: MgO:LiNbO 3 (2.70 × 10 −20 cm 2 for σ -polarization) [18] , but also larger than that of Nd 3+ :YAG (7.30 × 10 −20 cm 2 ) [15] . The Judd–Ofelt theory has become standard tool for evaluating the spectroscopic parameters of rare-earth ions in crystals and glasses [19,20] . The oscillator strength parameters Ω t ( t = 2, 4, 6) can be calculated from the absorption spectra, and then the spontaneous-emission probabilities, fluorescence branching ratios and radiative lifetimes of the excited manifolds can be estimated. The line strengths of the electric-dipole transition from the ground state 4 I 9/2 to the excited multiplet can be extracted from the corresponding room temperature absorption spectra by the following formula: (4) S q meas ( J → J ′ ) = 3 hc ( 2 J + 1 ) 8 π 3 e 2 λ ¯ N 9 n ( n 2 + 2 ) 2 ∫ α q ( λ ) d λ where the subscript q indicates the polarization of spectra, N is the concentration of Nd 3+ ions in the sample (1.07 × 10 20 ions/cm 3 ), λ ¯ is the average wavelength of the absorption band, n is the refractive index, α q ( λ ) is the absorption coefficient and ∫ α q ( λ ) d λ is the integrated absorption coefficient for each absorption band. The refractive indexes n are obtained from the Sellmeier equation established elsewhere for BaCaBO 3 F crystal [13] : (5) n o 2 = 2.71455 + 0.01871 λ 2 λ 2 - 0.01730 - 0.00906 λ 2 (6) n e 2 = 2.55994 + 0.01599 λ 2 λ 2 - 0.01752 - 0.00528 λ 2 where λ represents the wavelength (unit: μm). In the subsequent calculation, the values of ordinary and extraordinary refractive indices of the crystal are used for the σ - and π -polarized spectra, respectively. In the framework of the Judd–Ofelt theory, the line strength of the electric-dipole transition can also be expressed as (7) S q cal ( J → J ′ ) = ∑ t = 2 , 4 , 6 | 〈 4 f n [ α SL ] J ‖ U ( t ) ‖ 4 f n [ α ′ S ′ L ′ ] J ′ 〉 | 2 where ‖ U ( t ) ‖ are the reduced matrix elements of unit tensor operators calculated by Carnall et al. [21] , which are almost insensitive to the ion environment. By a least-square fitting between Eqs. (4) and (7) , the intensity parameters Ω t ( t = 2, 4, 6) were obtained. The effective intensity parameters, which are defined as Ω eff = ( Ω π + 2 Ω σ )/3, are also acquired. Making use of Eq. (7) and the obtained Ω t values, the calculated line strengths S cal can be determined. The values of experimental and calculated line strengths are listed in Table 1 . In Table 2 , the Judd–Ofelt parameters that result from the analysis mentioned above are compared with the values obtained for several laser crystals containing Nd 3+ . The spectroscopic quality factor, X = Ω 4 / Ω 6 , first introduced by Kaminskii and Li [28] , is also an important laser characteristic in predicting the stimulated emission in laser active medium. For Nd 3+ ion, the intensities of the 4 F 3/2 → 4 I 9/2 , 4 I 11/2 , 4 I 13/2 and 4 I 15/2 transitions are dependent only on the Ω 4 and Ω 6 , because the matrix elements of the operator U ( λ ) of rank 2 between the 4 F 3/2 and 4 I J manifolds are equal to zero [29] . Thus, the comparison between branching ratios of the 4 F 3/2 → 4 I 9/2 and 4 F 3/2 → 4 I 11/2 transitions can be related to the spectroscopic quality parameter X . With the reduction of X , the branching ratio of the 4 F 3/2 → 4 I 11/2 transition increases, whereas that of the 4 F 3/2 → 4 I 9/2 decreases. The value of X for the Nd 3+ :BaCaBO 3 F is determined to be 0.83, which is comparable to that of Nd 3+ :YVO 4 (0.80) [23] and is much lower than those of the Nd 3+ :Ca 4 GdO(BO 3 ) 4 (1.74) [5] , Nd 3+ :Ca 4 YO(BO 3 ) 4 (1.92) [6] and Nd 3+ :YAl 3 (BO 3 ) 4 (1.62) [26] . It should be pointed out that a low X value is favorable for the 4 F 3/2 → 4 I 11/2 transition around 1060 nm but disadvantageous for the 4 F 3/2 → 4 I 9/2 transition around 900 nm. To justify the results obtained, the root-mean-square deviation of the experiment and calculation oscillator line strengths is introduced, which is defined by: (8) rms Δ S q = ∑ i = 1 N ( S q meas - S q cal ) 2 N - 3 where N is the number of experimental absorption bands. The measurement of the relative error is given by: (9) Rms error = rms Δ S / rms S × 100 % where (10) rms S = ∑ 1 N S meas 2 / N The values of the rms error are 8.0% and 5.2% for π - and σ -polarization, respectively, which are in the typical error range of J–O fitting and indicate good agreement between the calculated and experimental results. Once the J–O intensity parameters have been obtained, the spontaneous emission probability A from the initial multiplet 4 F 3/2 to the terminal multiplets 4 I J′ ( J ′ = 9/2, 11/2, 13/2 and 15/2) can be calculated by (11) A ( J → J ′ ) = 64 π 4 e 2 3 h ( 2 J + 1 ) λ ¯ 3 n ( n 2 + 2 ) 2 9 ∑ t = 2 , 4 , 6 Ω t | 〈 4 F 3 / 2 ‖ U t ‖ 4 I J ′ 〉 | where the deduced emission transition matrix elements | 〈 ‖ U t ‖ 〉 | were given by Kaminski et al. [29] and λ ¯ is the mean wavelength of the fluorescence band. Then, the fluorescence branching ratio β is defined as (12) β q ( J → J ′ ) = A q ( J → J ′ ) ∑ J ′ A q ( J → J ′ ) The radiative lifetime of a multiplet τ r is the reciprocal of the total spontaneous-emission probabilities from the 4 F 3/2 multiplet. For an anisotropic crystal, the total spontaneous-emission probabilities are expressed as: (13) A total = ∑ q ∑ J ′ A q ( J → J ′ ) 3 (14) τ r = 1 A total Thus, the radiative lifetime of the 4 F 3/2 multiplet of Nd 3+ ions in the BaCaBO 3 F crystal is 215.73 μs. The calculated spontaneous-emission probabilities, radiative lifetimes, and branching ratios for the main emission transitions of Nd 3+ in BaCaBO 3 F crystal, are summarized in Table 3 . 3.4 Fluorescence spectra and emission cross-sections Fig. 3 displays the fluorescence spectra of Nd 3+ :BaCaBO 3 F crystal at room temperature, which was excited by the 813 nm laser radiation. Three emission bands correspond to the 4 F 3/2 → 4 I 9/2 , 4 F 3/2 → 4 I 11/2 and 4 F 3/2 → 4 I 13/2 transitions, respectively. The polarized stimulated emission cross-sections at various wavelengths was estimated from the polarized fluorescence spectra by the Füchtbauer–Ladenburg (F–L) formula in order to ascertain the potentialities for research and industrial applications [30] (15) σ em = λ 5 β 8 π cn 2 1 τ r I ( λ ) ∫ λ I ( λ ) d λ where n is the refractive index, c is the speed of light, I ( λ ) is the relative fluorescence intensity, τ r is the radiative lifetime, β is the fluorescence branching ratio. Thus, the stimulated emission cross-sections of Nd 3+ :BaCaBO 3 F crystal were obtained, as depicted in Fig. 4 . The values at peak fluorescence wavelengths are listed in Table 4 . The blue laser is of practical interest in various fields, such as high-density optical data storage, photo therapy, medical diagnostic, etc. A very promising modality to obtain lasers in the blue spectral range is the frequency doubling of the infrared 900–950 nm range emission from the 4 F 3/2 → 4 I 9/2 transition of Nd 3+ transition. Short fundamental laser wavelengths around 900 nm require Nd 3+ -activated crystals whose splitting between 4 F 3/2 emitting level and 4 I 9/2 ground level is large [31] , for instance, the 4 F 3/2 → 4 I 9/2 laser emission occurs at 946 nm for Nd 3+ :YAG in which an efficient quasi-three-level laser emission has been realized [32] . It can be observed from Fig. 3 that Nd 3+ :BaCaBO 3 F crystal exhibited strong signals at 943 nm. The stimulated emission cross-sections were determined to be 1.79 × 10 −20 cm 2 at 943 nm for σ -polarization, which are slightly smaller than those of Nd 3+ :YAG (3.7 × 10 −20 cm 2 ) and Nd 3+ :YVO 4 (4.8 × 10 −20 cm 2 for π -polarization) [33] . It should be noted that the emission wavelength at 943 nm is far way from the reabsorption region around 880 nm. Therefore, the crystal deserves further investigations on laser operation around 900 nm. The 4 F 3/2 → 4 I 11/2 transition is the most important laser channel of Nd 3+ ions. The FWHMs of the emission band are 6.3 and 5.9 nm for π - and σ - polarization polarizations, respectively. The peak stimulated emission cross-sections were calculated to be 7.22 × 10 −20 cm 2 and 15.21 × 10 −20 cm 2 at 1068 nm for π - and σ -polarization, respectively, which is much larger those of Nd 3+ :YAl 3 (BO 3 ) 4 (9.7 × 10 −20 cm 2 for σ -polarization) [8] , Nd 3+ :Ca 4 GdO(BO 3 ) 4 (4.21 × 10 −20 cm 2 for E//X) [5] and Nd 3+ :Gd 2 (MoO 4 ) 3 (11.0 × 10 −20 cm 2 for σ -polarization) [24] . Nd 3+ :BaCaBO 3 F shows some advantages of high absorption and emission cross-sections, so the crystal may be regarded as a promising solid-state infrared laser material for diode-laser pumping. The 4 F 3/2 → 4 I 13/2 laser channel of Nd 3+ ions provides a way of realizing solid-state infrared lasers emitting in the 1.3 μm range, and these laser sources have important applications in remote sensing, guided optical communications and soft human tissue applications. The peak stimulated emission cross-sections were calculated to be 7.57 × 10 −20 cm 2 at 1341 nm for σ -polarization, respectively, which is the same order as Nd 3+ :YAG (9.0 × 10 −20 cm 2 ) and Nd 3+ :KGd(WO 4 ) 2 (7.6 × 10 −20 cm 2 ) [34] . 3.5 Fluorescence lifetime and quantum efficiency Fig. 5 presents the luminescence decay curve of 4 F 3/2 multiplet at room temperature. The linear relationship displays single exponential behavior of the fluorescence decay, and the fluorescence lifetime can be obtained from the slope of the fitting line k , i.e. τ f = −1/2.303 k . Thus, the fluorescence lifetime of 4 F 3/2 multiplet was calculated to be 57.7 μs at room temperature, which is the typical value of the fluorescence lifetime in borate crystal matrix [6,25–27] . Then the fluorescence quantum efficiency η ( η = τ f / τ r ) is derived as 26.8%, which is much lower than those of Nd 3+ :MgO:LiNbO 3 (94.0%) [18] and Nd 3+ :Gd 2 (MoO 4 ) 3 (95.0%) [24] . The low quantum efficiency means that the nonradiative transition rate in the Nd 3+ :BaCaBO 3 F crystal is high. Generally, the cross relaxation between Nd 3+ ions and the multiphonon relaxation caused by the interactions between the Nd 3+ ions and the host, are the main reasons for the nonradiative transition. Considering the relatively low Nd 3+ concentration (1.1 at.%) in the crystal, the effect of the cross relaxation could be neglected. According to the so-called energy gap law [35] , the multiphonon nonradiative transition rate mainly depends on the energy gap between the excited state and the next lower level and the highest phonon energy of the host crystal. The smaller the energy gap and the larger the highest phonon energy is, the higher multiphonon nonradiative transition rate is. Since the energy gap between the 4 F 3/2 emitting level and the next-lower state 4 I 15/2 (∼5000 cm −1 ) is narrow with respect to the maximum phonon energy of the host lattice (∼1300 cm −1 ), the nonradiative transition rate in Nd 3+ :BaCaBO 3 F is relatively high, which most likely resulting in a low fluorescence quantum efficiency. However, it is interesting to observe that the fluorescence quantum efficiency η of Nd 3+ :BaCaBO 3 F crystal is remarkably higher than those of other borates, e.g. Nd 3+ :GdAl 3 (BO 3 ) 4 (18.7%) [25] , Nd 3+ :YAl 3 (BO 3 ) 4 (18.0%) [6] , Nd 3+ :Ca 4 GdO(BO 3 ) 3 (15.0%) [5] and Nd 3+ :Ca 4 YO(BO 3 ) 3 (15.0%) [6] . In Ref. [36] , the decreasing of the multiphonon nonradiative relaxation probability with reduction of the contents of oxyboron (BO 3 ) complexes in structure of crystals is observed. The fact that the contents of oxyboron (BO 3 ) complexes in BaCaBO 3 F crystal is lower than those in other borates mentioned above, may account for the lower multiphonon nonradiative relaxation rate and then higher luminescence quantum output. 3.6 Preliminary analysis of self-frequency doubling properties As a non-linear optical crystal, the BaCaBO 3 F crystal can be used for frequency doubling of 1064 nm of Nd:YAG laser radiation for type I and type II phase matching (PM) [12,13] . In addition, BaCaBO 3 F can achieve third harmonic generation by sum frequency generation (1064 + 532 → 355 nm) [13] . According to the Sellmeier equation [13] , the phase matching angles were calculated to be 36.8° for type I and 56.0° for type II second-harmonic (SH) generation of 1068 nm light. We also calculated the phase matching angles for type I and type II SH generation of 943 nm light to be 41.5° and 66.5°. Table 5 presents some non-linear and spectroscopic characteristics of Nd 3+ :BaCaBO 3 F and several well-known self-frequency doubling crystals for comparison. As can be seen, the absorption and emission cross-sections are much higher for σ -polarization than that for π -polarization, which is an advantage of Nd 3+ :BaCaBO 3 F as a SFD crystal. For Nd 3+ :BaCaBO 3 F crystal is a uniaxial negative birefringence crystal, the fundamental radiation should be σ polarized in order to obtain type I SFD. Thus, pumping with an ordinary ( σ polarized) beam, which is free from walk-off, will lead to a better spatial overlap between the pump and laser modes than by pumping with an extraordinary beam [8] . Self-absorption of second-harmonic (SH) radiation is an important process that may lead to a crystal temperature increase affecting the conversion efficiency [8] . The absorption at 471 nm is negligible, so the blue radiation, obtained by SFD of the infrared emission corresponding to the 4 F 3/2 → 4 I 9/2 transition of Nd 3+ , would not be affected by the self-absorption. The absorption cross-sections is 1.53 × 10 −20 cm 2 at 534 nm for σ -polarization, which is much larger than those of Nd 3+ :YAl 3 (BO 3 ) 4 (0.31–0.55 × 10 −20 cm 2 for σ -polarization) [8,16] and Nd 3+ :Ca 4 GdO(BO 3 ) 4 (0.27 × 10 −20 cm 2 for E//X) [5] . Therefore, self-absorption has become the most probable obstacle to realize SFD output for Nd 3+ :BaCaBO 3 F crystal at 534 nm wavelength. An optimum Nd 3+ concentration and crystal length must be chosen carefully for possible further laser operation. The optimum Nd 3+ concentration for Nd 3+ :BaCaBO 3 F crystal can be determined by taking fully into consideration absorption efficiency, concentration quenching and the influence of Nd 3+ doping on the non-linear susceptibility as well as SH self-absorption effect [8] . The optimal crystal length should be a balance between minimizing the SH absorption losses, while maximizing the pump absorption and the useful phase matching pathlength [10] . 4 Conclusions In this work, we attempt to explore the possibility of Nd 3+ :BaCaBO 3 F as a new infrared and visible laser crystal, based on spectroscopic measurements and data on linear and non-linear optical properties. Through the analysis of polarized absorption spectra in the framework of the Judd–Ofelt theory, the main spectroscopic characteristics have been determined. The emission cross-sections have been calculated to be 7.22 × 10 −20 cm 2 and 15.21 × 10 −20 cm 2 at 1068 nm for π - and σ -polarization using the Füchtbauer–Ladenburg (F–L) equation. The fluorescence lifetime of 4 F 3/2 manifold is equal to 57.7 μs, and the quantum efficiency is 26.8%, which is higher than those of most of other borates. The results indicate that Nd 3+ :BaCaBO 3 F crystal may be regarded as a efficient infrared laser crystal material for diode-laser pumping. However, an appreciable self-absorption of the SH wavelength could impose some restrictions on its SFD applications. Compared with green radiation, SFD of the fundamental laser line at 943 nm is more promising due to no SH self-absorption, proper birefringence and large splitting of 4 I 9/2 multiplet. Acknowledgements This project was supported by the Nature Science Foundation of Education Department of Anhui Province ( KJ2010B203 and KJ2010B205 ) and the Science Foundation of Shandong Province ( ZR2009BM041 ). Appendix A Supplementary material Supplementary data associated with this article can be found, in the online version, at doi:10.1016/j.optmat.2010.11.021 . Appendix A Supplementary material Supplementary data 1 Table S1. The Data of X-ray powder diffraction for Nd 3+ :BaCaBO 3 F crystal. References [1] T.Y. Fan A. Cordova-Plaza M.J.F. Digonnet R.L. Byer H.J. Shaw J. Opt. Soc. Am. B 3 1986 140 148 [2] J. Bartschke R. Knappe K.-J. Boller R. Wallenstein IEEE J. Quantum Electron. 33 1997 2295 2300 [3] D. Jaque J. Capmany J. García Solé Z.D. Luo A.D. Jiang J. Opt. Soc. Am. B 15 1998 1656 1662 [4] B. Lu J. Wang H. Pan M. Jiang J. Appl. Phys. 66 1989 6052 6054 [5] F. Mougel G. Aka A. Kahn-Harari H. Hubert J.M. Benitez D. Vivien Opt. Mater. 8 1997 161 173 [6] D.A. Hammons M. Richardson B.H.T. Chai A.K. Chin R. Jollay IEEE J. Quantum Electron. 36 2000 991 999 [7] A. Brenier D. Jaque A. Majchrowski Opt. Mater. 28 2006 310 323 [8] D. Jaque J. Capmany J.A. Sanz García A. Brenier G. Bouglon J. García Solé Opt. Mater. 13 1999 147 157 [9] D.A. Keszler A. Akella K.I. Schaffers T. Alekel III Mater. Res. Soc. Symp. Proc. 329 1994 15 22 [10] K.I. Schaffers L.D. Deloach S.A. Payne IEEE J. Quantum Electron. 32 1996 741 748 [11] G.C. Zhang H.J. Liu X.A. Wang F.D. Fan P.Z. Fu J. Cryst. Growth 289 2006 188 191 [12] X. Wang G.C. Zhang Y. Zhao F.D. Fan H.J. Liu P.Z. Fu Opt. Mater. 29 2007 1658 1661 [13] K. Xu P. Loiseau G. Aka J. Cryst. Growth 311 2009 2508 2512 [14] Y. Sato T. Taira IEEE J. Quantum Electron. 11 2005 613 620 [15] J. Lu M. Prabhu J. Song C. Li J. Xu K. Ueda A.A. Kaminskii H. Yagi T. Yanagitani Appl. Phys. B 71 2000 469 473 [16] S.J. Zhang Z.X. Cheng J.R. Han G.Y. Zhou Z.S. Shao C.Q. Wang Y.T. Chow H.C. Chen J. Cryst. Growth 206 1999 197 202 [17] G.F. Wang Z.B. Lin Z.S. Hu T.P.J. Han H.G. Gallagher J-.P.R. Wells J. Cryst. Growth 233 2001 755 760 [18] R. Burlot R. Moncorgé H. Manaa G. Boulon Y. Guyot J. García Soléand D. Cochet-Muchy Opt. Mater. 6 1996 313 330 [19] B.R. Judd Phys. Rev. 127 1962 750 761 [20] G.S. Ofelt J. Chem. Phys. 37 1962 511 520 [21] W.T. Carnall P.R. Fields K. Rajnak J. Chem. Phys. 49 1968 4424 4442 [22] W.F. Krupke IEEE J. Quantum Electron. QE-7 1971 153 159 [23] T.S. Lomheim L.G. DeShazer J. Appl. Phys. 49 1978 5517 5522 [24] D. Jaque J. Findensein E. Montoya J. Capmany A.A. Kaminskii H.J. Eichler J. García Solé J. Phys.: Condens. Matter 12 2000 9699 9714 [25] G.F. Wang J. Opt. Soc. Am. B 18 2001 173 175 [26] D. Jaque J. Capmany Z.D. Luo J. García Solé J. Phys.: Condens. Matter 9 1997 9715 9729 [27] R. Guo Y.C. Wu P.Z. Fu F.L. Jing J. Opt. Soc. Am. B 22 2005 831 834 [28] A.A. Kaminskii L. Li Phys. Status Solidi A 26 1974 593 598 [29] A.A. Kaminskii G. Boulon M. Buoncristiani B. Di Bartolo A. Kornienko V. Mironov Phys. Status Solidi A 141 1994 471 494 [30] B.F. Aull H.P. Jenssen IEEE J. Quantum Electron. 18 1982 925 930 [31] C. Varona P. Loiseau G. Aka B. Ferrand Proc. SPIE 6190 2006 619004 [32] P. Zeller P. Peuser Opt. Lett. 25 2000 34 36 [33] C. Czeranowsky M. Schmidt E. Heumann G. Huber S. Kutvoi Y. Zavartsev Opt. Commun. 205 2003 361 365 [34] O. Musset J.P. Boquillon Appl. Phys. B 64 1997 503 506 [35] F. Auzel F. Pellé Phys. Rev. B 55 1997 11006 11009 [36] V.A. Lebedev, I.V. Voroshilov, B.V. Ignatiev, A.N. Gavrilenko, V.F. Pisarenko, A.V. Saakyan, in: Advanced Solid State Lasers, OSA Technical Digest Series, Optical Society of America, 2000, MB6. [37] A.A. Kaminskii S.N. Bagaev K. Ueda A.A. Pavlyuk M. Musha Quantum Electron. 27 1997 657 658 [38] A. Brenier C.Y. Tu M.W. Qiu A.D. Jiang J.F. Li B.C. Wu J. Opt. Soc. Am. B 18 2001 1104 1110
更多查看译文
关键词
Solid-state laser materials,Fluoborate,Optical microscopy,Frequency doubling
AI 理解论文
溯源树
样例
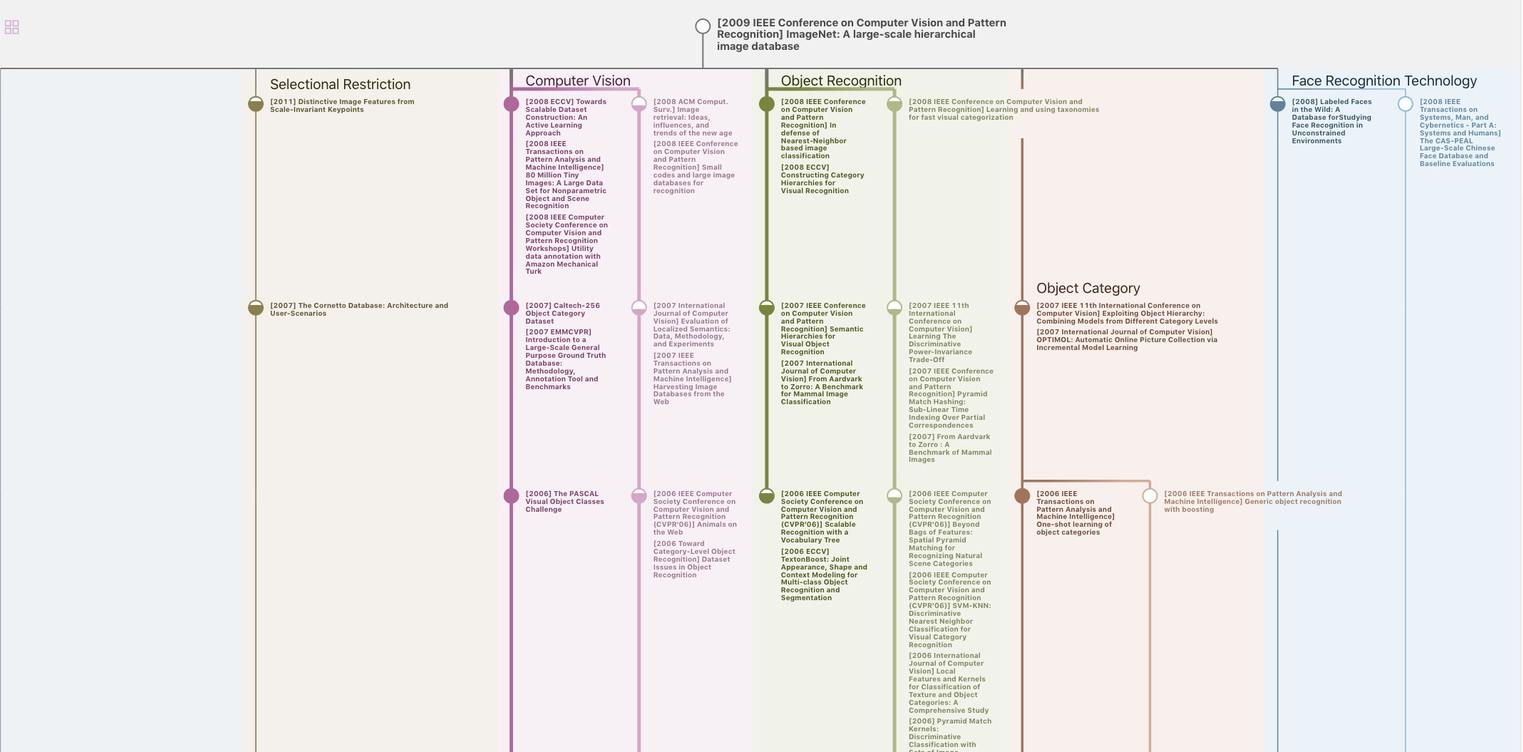
生成溯源树,研究论文发展脉络
Chat Paper
正在生成论文摘要