Variability Of Prostate Brachytherapy Preimplant Dosimetry: A Multi-Institutional Analysis.
Brachytherapy(2005)
摘要
Results The mean planning target volume to prostate volume ratio varied dramatically (mean 1.29, range 0.99–1.76) with the target length ranging from 3.5 to 4.5 cm. Although the prostate V 100 was >95% in all cases, the V 150 ranged from 29.9% to 92.1% and the V 200 from 6.72% to 52.5%. The urethral V 100 was 100% in all cases with six of the eight brachytherapists limiting the UV 150 to <3%. However, the median urethral dose varied by up to 50%. Treatment margins also varied significantly (average 3.98 mm, range 0.32–7.68 mm). All brachytherapists used extracapsular seeds with five implanting >25% of the seeds in extracapsular locations (range 6.4–58.2%). In addition, significant variability existed in the number of needles, number of seeds, and seed strength. Conclusions This study highlights the substantial variability that exists regarding target volume, seed strength, dose homogeneity, treatment margins, and extracapsular seed placement, although prostate brachytherapy prescription doses are uniform. The standardization of preimplant dosimetry is essential for meaningful multi-institutional comparisons of biochemical outcomes and morbidity. Keywords Prostate Brachytherapy Dosimetry Pd-103 I-125 Introduction Despite the widespread acceptance of prostate brachytherapy as a curative treatment for clinically localized prostate cancer, a paucity of evidence-based data has accumulated regarding the relationships between planning parameters, biochemical control rates, and morbidity profiles. Although multiple permanent prostate brachytherapy seed planning philosophies are currently used, definitive recommendations for preimplant dosimetry have not been formulated. The absence of broad-based guidelines has made multi-institutional comparison of biochemical and quality of life outcomes questionable. Quality is easy to conceptualize, but difficult to quantitate. It is universally accepted that an adequate implant should encompass the target volume, but there is no consensus as to what represents the target volume. In addition, substantial variability exists regarding seed strength, choice of isotope, seed distribution, dose homogeneity, treatment margins, and normal tissue tolerances (1, 2) . These planning differences along with the imprecision of intraoperative execution may account for some of the reported institutional differences in biochemical control rates and morbidity (3–6) . To compare prostate brachytherapy preimplant dosimetry, eight experienced prostate brachytherapists submitted Pd-103 and I-125 plans for an identical ultrasound volumetric study for central review. Methods and materials Eight prostate brachytherapists with extensive experience with Pd-103 and I-125 monotherapy were invited to participate in the study. All brachytherapists received the same transrectal ultrasound volumetric study with the prostate contoured on each 5 mm slice (Siemens Medical Systems, Issaquah, WA) and instructions to generate four plans (Pd-103 monotherapy, prescription dose 125 Gy ABS-2000; I-125 monotherapy, prescription dose 145 Gy TG-43; Pd-103 boost, prescription dose 90 Gy ABS-2000; I-125 boost, prescription dose 110 Gy TG-43). The prostate volume was 30.1 cm 3 with a prostate length of 3.50 cm. A urethral catheter was in place at the time of ultrasonography for urethral identification. For the monotherapeutic plans, the clinical history consisted of a patient with clinical Stage T1c, prostate-specific antigen (PSA) 6.0 ng/mL, Gleason score 6 (3 + 3), and two of eight biopsies positive for malignancy without evidence of perineural invasion. For the boost plans, the patient profile included clinical Stage T1c, PSA 12.0 ng/mL, Gleason score 7 (4 + 3), and two of eight biopsies positive with evidence of perineural invasion. The brachytherapy planning instructions were as follows: “If your planning approach utilizes a defined target volume (prostate plus margin) that you normally draw on the ultrasound, please do. If you normally do not define such a volume, do not change your approach. Please generate each of the four requested plans as if this were a patient in your clinic. Regardless of whether or not you normally report urethral doses, please do so. For this study, the only acceptable seeds are Theraseed Model 200 (Theragenics Inc., Buford, GA) and Oncura Oncoseed Model 6711 (Oncura, Plymouth Meeting, PA).” Seven of the eight investigators generated plans using Variseed (Varian Medical Systems, Palo Alto, CA), whereas the other brachytherapist used Rosses Medical Systems (Carmichaels, PA). The plans generated on the Rosses system had their seed coordinates and planning target volumes (PTV) redigitized into Variseed. The calculation algorithms and seed parameters used in planning were recommended by the American Association of Physicists in Medicine Task Group No. 43 (7) . Central dosimetric review included evaluation of target volume, target to prostate ratio, target length, number of needles, seed activity, number of seeds, total activity, total activity divided by target planning volume, number of extracapsular seeds including seminal vesicle and infra-apical seeds (defined as seeds planned inferior to the apex of the prostate gland), and average treatment margins (defined as the perpendicular distance between the ultrasound-determined prostate capsule and the 100% isodose line). The dosimetric treatment margins were determined by a software package under development by Variseed. Margins were determined at eight angles (every 45°) on every transverse prostate ultrasound slice. The software was not designed to measure superior or inferior margins. Cumulative dose–volume histograms (DVHs) and natural DVHs were determined for each plan (8) . The prostate dosimetric evaluation was defined by the volume of the gland receiving 100%, 150%, 200%, and 300% of the prescription dose (V 100 /V 150 /V 200 /V 300 ) and the minimum dose received by 100%, 90%, and 50% of the prostate gland (D 100 /D 90 /D 50 ). Urethral dosimetry was defined in terms of the volume of the urethra receiving 100%, 150%, and 200% of the prescribed dose (UV 100 /UV 150 /UV 200 ) and the minimum dose received by 90% and 50% of the urethra (UD 90 /UD 150 ). Robustness of the plans to random seed perturbations was tested by applying a standard deviation of 2 mm in the lateral and anterior–posterior directions ( x , y ) and a standard deviation of 3 mm in the superior–inferior direction ( z ) (9) . Prostate and urethral quality parameters ( V xx and D yy ) were recorded for each perturbation as were dosimetric margins at selected angles on each of the eight prostate slices. Pearson correlation coefficients and their statistical significance between dosimetric margins and other implant parameters were determined using SPSS 12.0 (SPSS Inc., Chicago, IL). Changes in the mean values of all dosimetric and marginal parameters after the 100 perturbation cycles were also tested for significance and correlation with all the initial dosimetric and marginal parameters. Comparisons between brachytherapists and modalities used a linear mixed model analysis where the data may exhibit correlated and nonconstant variability. Of the numerous models tested, a first-order autoregressive covariate structure provided the best fit to the data for most clinical and dosimetric parameters and therefore was used throughout. Results Table 1 summarizes the volumetric planning parameters for Pd-103 and I-125 monotherapy and boost plans. An explicit PTV was drawn by six of eight investigators. The mean PTV to prostate ratio was 1.37 among those six with a ratio of 1.29 for the entire group. The drawn PTV to prostate volume ratio varied dramatically from brachytherapist to brachytherapist, ranging from 0.99 to 1.76. The target length was taken to be the distance from the most superior seed slice to the most inferior seed slice and was the same as the transverse ultrasound prostate length (3.5 cm) in the plans of three investigators. Two investigators added seeds both superior and inferior to the ultrasound (US) prostate, and the rest added seed planes either superiorly or inferiorly so that the target length also varied from 3.5 to 4.5 cm. The number of needles, needle loading characteristics, seeds, and seed strength showed considerable variation as detailed in Table 2 . Within each institution, planning dosimetric quality parameter trends were consistent for monotherapy and boost plans and as such, subsequent tables summarize data only for monotherapy. Although there was a wide range in the number of needles within each approach (a high-to-low ratio of 2.1), the mean number of needles across the four modalities differed by less than one. Specially loaded needles are those multiseed needles that differ in any way from the regular 1 cm center-to-center seed spacing. The designation characterized either extra spacing between seeds or the presence of back-to-back seeds. Although only two brachytherapists used single seed needles, all brachytherapists used specially loaded needles ranging from less than 10% of the total number of needles to nearly 90%. The seed strength for boost therapy was typically about 20% lower than that for monotherapy, although one brachytherapist (D) used the same strength for monotherapy and boost so that the range of seed strengths (high/low) for a given modality was as large as 2.9 for I-125 boost. Total seed strength was about 25% less for boost therapy compared to monotherapy with a range within each modality (high/low) of 1.6 in I-125 and Pd-103 boost to 1.8 in Pd-103 monotherapy. One investigator (E) used the same number of needles and seeds for all four plans by maintaining a constant seed strength to prescription dose ratio. For the entire group, on average two to three fewer seeds were used in boost plans compared to those used in monotherapy. The total activity per cm 3 of planned target volume also displayed significant high/low variation (as high as 1.9–2.1). Table 2 delineates large variations in the use of extracapsular seeds. Striking differences were discerned in both absolute number and percent of planned total extracapsular seeds along with marked differences in the extracapsular seed placement. Six of the eight brachytherapists planned more than 25% of the seeds in extracapsular locations. However, only four of the eight brachytherapists planned seminal vesicle seeds with only three using infra-apical seeds. Of note, no seed was placed greater than 5 mm beyond the prostate capsule. The DVHs for Pd-103 and I-125 monotherapy plans are shown in Fig. 1 . All the curves depart from the 100% volume coverage at different dose levels and then diverge further because of differing slopes. Higher slopes expressed as %volume/%prescription dose at the D 50 level indicate greater dose homogeneity. For Pd-103, the slopes range from 0.54 (investigator D) to 1.63 (A) and for I-125 from 0.83 (D) to 2.86 (E). In all cases, urethral DVHs completely encompassed the urethra with the prescription dose. The DVHs for monotherapy plans are plotted in Fig. 2 . The slopes of the urethral DVHs at the D 50 level of the Pd-103 plans were quite steep, and the variation of dose at D 50 level was small (108–141% of prescription dose). The slopes at D 50 for I-125 were even steeper, but the spread of doses at D 50 level was somewhat greater (108–153% of prescription dose). Prostate and urethral monotherapeutic dosimetric parameters are summarized in Table 3 . A natural dose ratio (the natural prescription dose to the prescribed prescription dose) of 1.00 represents the most efficient use of dose, whereas higher and lower values reflect overdosing and underdosing of the prostate gland, respectively. The mean ratios were nearly ideal, whereas individual plans ranged from 1.15 for Pd-103 boost therapy to 0.82 for Pd-103 monotherapy. Coverage of the prostate in terms of V 100 was greater than 95% for all investigators, and urethral coverage in terms of UV 100 was 100% in all cases ( Table 3 ). The similarities, however, end at that point. Significant variations in V 150 with values as high as 86.0% and as low as 29.9% of prescription dose were noted in Pd-103 plans and from 92.1% to 31.7% of prescription dose in I-125 plans. A similarly large variation was seen in V 200 values, which ranged from as low as 6.7% to as high as 52.5%. Because of the relative seed strength and fall-off differences between Pd-103 and I-125, the average V 150 , V 200 , and V 300 , are notably higher for Pd-103 plans than I-125 plans. The boost therapy values of those three parameters were each slightly higher than those of their monotherapy counterparts due to the use of a higher seed strength to prescription dose ratio in boost plans compared to that in monotherapy plans. In terms of D 100 , only one Pd-103 plan and three I-125 plans had minimum prostate dose coverage that equaled or exceeded the prescribed dose for both monotherapy and boost with D 100 coverage as low as 67% of prescription dose ( Table 3 ). D 90 values demonstrated somewhat less variability with all institutions delivering at least 112% of prescription dose with a maximum of 151%. D 50 values represent the median dose to the gland. Consistent with significant inconsistencies in dose homogeneity (V 150 ), the high/low D 50s varied by as much as 1.50. In terms of urethral dosimetry, all institutions delivered 100% of the prescribed dose to the urethra with six of eight Pd-103 plans demonstrating a urethral V 150 less than 3% for Pd-103 and I-125 ( Table 3 ). Consistent with the prostate D 90 , the urethral D 90 revealed somewhat less variability, whereas the D 50 (representing the median urethral dose) varied significantly from investigator to investigator with differences approaching 50%. Selected slices from a composite isodose plan incorporating all seeds from all brachytherapists for Pd-103 monotherapy are shown in Fig. 3 . The 660 seeds placed by individual investigators resulted in 376 unique seed positions, and the maximum redundancy at any position was five seeds in four instances. One investigator used transverse needle, and longitudinal seed offsets were adjusted for purposes of the composite plan so that seeds appeared on the nearest regular grid coordinate. Investigators with the two highest number of unique seed locations were C and A with 58 and 56 positions, respectively. Investigators whose plans resulted in the fewest number of unduplicated positions were H and F with 5 and 9 positions, respectively. There were 118 duplicated positions with the most common pairings between investigators E and F (17 positions), G and H (16 positions), and A and D (14 positions). Of the 57 triplicate positions, the combination of investigators B, E, and F accounted for 32 occurrences. These three brachytherapists used regular 1 cm spacing between seeds in most needles and began many of their source trains 5 mm superior to the base ( Table 2 ). Monotherapy dosimetric treatment margins (defined as the perpendicular distance from the prostate capsule to the 100% isodose line) measured at eight angles on each of the eight slices are listed in Table 4 . The overall mean margin was 3.98 ± 2.64 mm. The more rapid dose fall-off with distance for Pd-103 compared with that of I-125 resulted in a trend for smaller margins in Pd-103. In boost plans, a trend for larger margins was noted because of a larger relative seed strength. As expected, the differences between brachytherapists were pronounced. For example, in Pd-103 monotherapy, the mean treatment margin was 3.81 mm with a range of 1.13–7.68 mm. For I-125, the range of treatment margins was similarly pronounced with a mean of 4.11 mm (range 0.32–7.49 mm). Table 5 lists coefficients of variation (CV) as the standard deviation/mean (as a percentage) for both investigators and treatment approaches. These coefficients indicate the degree of consistency of a given parameter across modalities for each investigator and across investigators for each modality. Negative values in the dosimetric margin parameters arose whenever a mean margin was negative. Parameters with the lowest CV are those that the brachytherapists tended to consider more important to maintain within a tightly constrained individual range. These crucial parameters include prostate V 100 and D 90 and urethral UD 50 and UD 10 . Dosimetric margins were not well controlled either by intent or because of difficulties in maintaining a standard. Except for a few cases, the consistency of the individual brachytherapist was greater across modalities than across investigators for each modality. The robustness of plans to random perturbations in seed position was tested by applying a 2 mm standard deviation to transverse seed coordinates and 3 mm SD to longitudinal coordinates. The mean percentage changes in dosimetric and marginal parameters after 100 perturbations are listed in Table 6 for monotherapy Pd-103. All plans were quite stable to perturbations, and the calculated changes are likely to be of no clinical consequence. Of the mean dosimetric and marginal parameters, only UV 150 and the posterior margin changed by more than 5%. In both cases, the initial unperturbed values for the overall mean and the individual brachytherapists were less than 0.02 cm 3 for UV 150 and less than 1 mm for the posterior margin ( Table 4 ) so that even inconsequential changes seem large as a percentage. Bivariate correlations between isodose margins and various treatment planning parameters were calculated to determine which factors have a significant effect on treatment margins ( Table 7 ). Significant positive correlations were found between treatment margins and PTV, extraprostatic seeds and needles, and V 90 , D 10 and UD 90 , UD 50 . Pearson correlation coefficients and significance values were also calculated between the original planning parameters and changes in dosimetric and marginal parameters after 100 seed perturbations. Table 8 shows only the original parameters and perturbed variables that had at least one significant bivariate correlation. What is most notable about Table 8 is that there were only 14 significant correlations out of a vast array of unperturbed–perturbed bivariate tests. It is not surprising that larger V 150 , D 100 , D 90 , and D 50 are associated with larger changes in V 100 , but it is surprising that parameters such as the number of seeds or extraprostatic seeds or the PTV/US volume ratio had no significant correlation. Discussion In general, four seed loading philosophies (uniform loading, peripheral loading, modified uniform loading, and modified peripheral loading) have been used in prostate brachytherapy with 75% of brachytherapists using a modified peripheral loading approach (10, 11) . Although it is universally accepted that an adequate implant should encompass the target volume, currently there is little consensus as to what represents the optimal target volume as illustrated in Table 1 . Sophisticated postimplant dosimetric analyses have demonstrated that biochemical disease-free survival and morbidity after prostate brachytherapy are related to specific source placement patterns and the dose gradients produced (4, 12–15) . Unfortunately, definitive recommendations for preimplant dosimetry that would maximize optimal postimplant dose distributions have not been formulated. Although no definitive far-reaching recommendations have been proposed for preimplant dosimetry, American Association of Physicists in Medicine Task Group No. 56 concluded that treatment plans be “designed to place seeds peripherally to improve dose homogeneity and to avoid unnecessary radiation damage to the urethra” (16) . Consistent with this statement, the ABS suggested that modified peripheral loading techniques be used to minimize the length of the urethra receiving 200% of prescription dose (17) . In contemporary prostate brachytherapy series, these specific recommendations are of little use. Even in this study, which demonstrated widely divergent urethral doses, doses >200% of prescription dose were not delivered to any segment of the urethra by any investigator. In this study, although prescription doses were uniform, substantial variability existed regarding the definition of target volume, seed strength, seed distribution, dose homogeneity, treatment margins, and the use of extracapsular seeds ( Tables 1 and 2 ). Substantial variations in treatment margins could potentially impact cure and/or brachytherapy-related morbidity (1, 2, 5, 6) . Despite these differences, all plans were highly resilient to seed misplacement ( Table 6 ). The rationale for periprostatic margins is based on pathologic measures of the probability of microscopic extracapsular disease and estimates that seed placement uncertainty is approximately 5 and 3 mm in the longitudinal and transverse directions, respectively (9, 18) . Previous detailed pathologic evaluations of radical prostatectomy series demonstrated that extracapsular extension is limited to within 5 mm of the prostate capsule in 99% of cases (19, 20) . In a previous ABS survey, Prete et al. (11) reported that 61% of brachytherapists added periprostatic margins with 5 mm being most common (range 1–10 mm). In this study, the PTV to prostate ratio and the extent of periprostatic treatment margins represented two of the most striking differences in implant philosophies ( Tables 1 and 4 ). Five of the eight brachytherapists used a target volume greater than the actual ultrasound volume with an overall planning target volume to prostate volume ratio of 1.29 (range 0.99–1.76). Monotherapy treatment margins ranged from 1.13 to 7.68 mm for Pd-103 and 0.32 to 7.49 mm for I-125. Generous periprostatic margins may necessitate the placement of periprostatic seeds. An ABS survey noted that 63% of brachytherapists implanted seeds in periprostatic locations (11) . In our series, all brachytherapists used extracapsular seeds with five of the eight brachytherapists implanting greater than 25% of seeds in extracapsular locations. However, only four of the eight brachytherapists used seminal vesicle seeds and three used infra-apical seeds. Of note, no investigator placed seeds greater than 5 mm beyond the prostate capsule. Although there was considerable controversy regarding target volume (i.e., the use of explicitly drawn planning treatment margins) and dose homogeneity, all brachytherapists generated a plan that resulted in a prostate V 100 >95%. Significant variation, however, was noted in dose homogeneity (V 150 ranged from 29.9% to 92.1% and V 200 from 6.7% to 52.5%; Table 3 ). Unfortunately, to date commonly accepted recommendations for dose homogeneity have not been formulated. The D 90 revealed somewhat less variability, however, the median dose (D 50 ) varied by as much as 50%. Identification of the urethra on the plan ensures the ability to use urethral sparing doses (100–150% of prescription dose) (1, 2, 21) . In an ABS survey, it was reported that two-third of the brachytherapists identified the urethra on the plan (11) . Volume parameters, such as UV 125 and UV 150 , and the dosimetric parameters, UD 10 and UD 25 , should be kept as small as possible (1, 2) . In the current study, all brachytherapists delivered >100% of prescription to the urethra with six brachytherapists limiting the UV 150 to <3%. However, the median urethral dose (D 50 ) varied significantly with differences approaching 50%. Although this study implies significant independent evolution of implant strategies, the explanation for these variations is beyond the scope of this study. However, it is likely that planning variations may be minimized with the inclusion of critical details regarding intraoperative execution (i.e., the accuracy of seed placement) and postimplant evaluation. Conclusions This study highlights that although prostate brachytherapy prescription doses are uniform, substantial variability exists regarding target volume, seed strength, dose homogeneity, treatment margins, and extracapsular seed placement. Standardization of preimplant dosimetry for the design of multi-institutional prospective randomized trials should facilitate multi-institutional comparison of biochemical and quality of life outcomes. Standardization of preimplant dosimetry should also help to ensure the reproducibility of published favorable outcomes. Acknowledgments The authors are grateful to Vrinda Narayana, PhD, Michael Sitter, RT(T), Zuofeng Li, PhD, Devi Naidoo, BS, RTT, and Siobhan O'Connor Hartsell, CMD for their assistance in the preparation of this manuscript. References [1] G.S. Merrick W.M. Butler Modified uniform seed loading for prostate brachytherapy: Rationale, design and evaluation Tech Urol 6 2000 78 84 [2] W.M. Butler G.S. Merrick J.H. Lief Comparison of seed loading approaches in prostate brachytherapy Med Phys 27 2000 381 392 [3] G.S. Merrick K.E. Wallner W.M. Butler Permanent interstitial brachytherapy for the management of carcinoma of the prostate gland J Urol 169 2003 1643 1652 [4] G.S. Merrick K.E. Wallner W.M. Butler Minimizing prostate brachytherapy-related morbidity Urology 62 2003 786 792 [5] A.V. D'Amico R. Whittington S.B. Malkowicz Biochemical outcome after radical prostatectomy, external beam radiation therapy, or interstitial radiation therapy for clinically localized prostate cancer JAMA 280 1998 969 974 [6] P.W. McLaughlin V. Narayana M. Kessler The use of mutual information in registration of CT and MRI datasets post permanent implant Brachytherapy 3 2004 61 70 [7] R. Nath L.L. Anderson G. Luxton Dosimetry of interstitial brachytherapy sources: Recommendations from the AAPM Radiation Therapy Committee Task Group No. 43 Med Phys 22 1995 209 234 [8] M.A. Moerland R. van der Laarse R.W. Luthmann The combined use of the natural and the cumulative dose–volume histograms in planning and evaluation of permanent prostatic seed implants Radiother Oncol 57 2000 279 284 [9] P.L. Roberson V. Narayana D.L. McShan Source placement error for permanent implant of the prostate Med Phys 24 1997 251 257 [10] B.R. Prestidge Radioisotopic implantation for carcinoma of the prostate: Does it work better than it used to? Semin Radiat Oncol 8 1998 124 131 [11] J.J. Prete B.R. Prestidge W.S. Bice A survey of physics and dosimetry practice of permanent prostate brachytherapy in the United States Int J Radiat Oncol Biol Phys 40 1998 1001 1005 [12] R.G. Stock N.N. Stone A. Tabert A dose response study for I-125 prostate implants Int J Radiat Oncol Biol Phys 41 1998 101 108 [13] L. Potters Y. Cao E. Calugaru A comprehensive review of CT-based dosimetry parameters and biochemical control in patients treated with permanent prostate brachytherapy Int J Radiat Oncol Biol Phys 50 2001 605 614 [14] G.S. Merrick W.M. Butler A.T. Dorsey Potential role of various dosimetric quality indicators in prostate brachytherapy Int J Radiat Oncol Biol Phys 44 1999 717 724 [15] G.S. Merrick W.M. Butler A.T. Dorsey The effect of prostate size and isotope selection on dosimetric quality following permanent seed implantation Tech Urol 7 2001 233 240 [16] R. Nath L.L. Anderson J.A. Meli Code of practice for brachytherapy physics: Report of the AAPM Radiation Therapy Committee Task Group No. 56 Med Phys 24 1997 1557 1598 [17] S. Nag D. Beyer J. Friedland American Brachytherapy Society (ABS) recommendations for transperineal permanent brachytherapy of prostate cancer Int J Radiat Oncol Biol Phys 44 1999 789 799 [18] A.W. Partin L.A. Mangold D.W. Lamm Contemporary update of prostate cancer staging nomograms (Partin tables) for the new millennium Urology 58 2001 843 848 [19] B.J. Davis T.M. Pisansky T.M. Wilson The radial distance of extraprostatic extension of prostate carcinoma Cancer 85 1999 2630 2637 [20] C. Sohayda P.A. Kupelian H.S. Levin Extent of extracapsular extension in localized prostate cancer Urology 55 2000 382 386 [21] W.M. Butler G.S. Merrick A.T. Dorsey Comparison of dose length, area, and volume histograms as quantifiers of urethral dose in prostate brachytherapy Int J Radiat Oncol Biol Phys 48 2000 1575 1582
更多查看译文
关键词
Prostate,Brachytherapy,Dosimetry,Pd-103,I-125
AI 理解论文
溯源树
样例
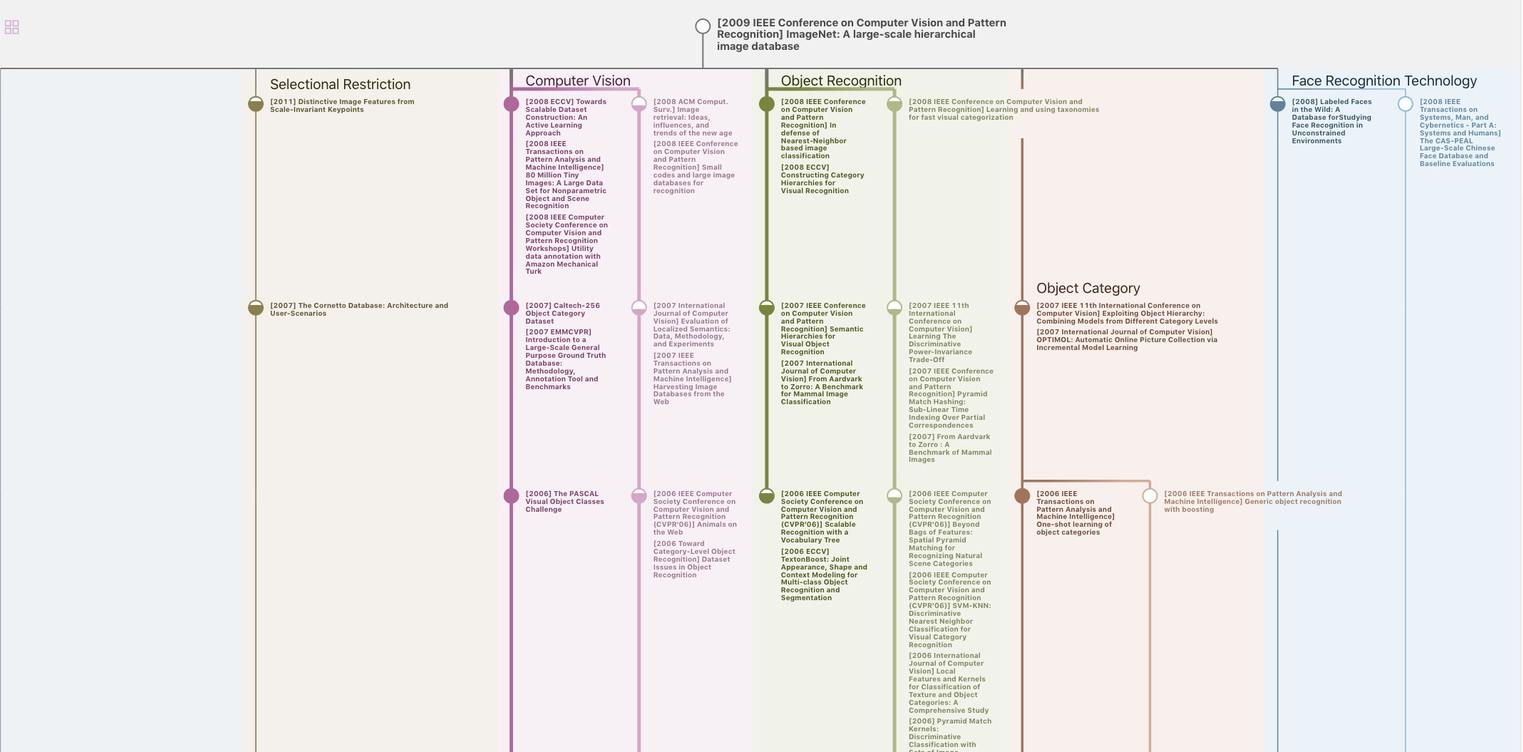
生成溯源树,研究论文发展脉络
Chat Paper
正在生成论文摘要