TGF-|[beta]|1 and TGF-|[beta]|2 expression after traumatic human spinal cord injury
SPINAL CORD(2008)
摘要
In contrast to the peripheral nervous system, lesioned axons within the adult mammalian central nervous system (CNS) do not undergo regeneration. The loss of function following traumatic spinal cord injury (SCI) is often permanent and results in a serious limitation of the patients' quality of life. Despite considerable progress in recent years, the underlying mechanisms responsible for the failure of axon regeneration after SCI remain only partially understood. Following SCI, the initial parenchymal damage is followed by a complex cascade of secondary events including a strong inflammatory reaction. The early inflammatory cascade is at least partially responsible for secondary tissue destruction and additional loss of function.1 The early phase of secondary parenchymal damage is followed by the removal of tissue debris. Finally, severe lesions become dominated by the deposition of scar tissue composed of connective tissue and fluid-filled cysts, surrounded by a dense astroglial scar.2 Multiple studies in experimental animals demonstrate that this scar represents a physical and molecular barrier that is important for isolating the lesion site from adjacent, intact spinal cord3 but is also crucial for the lack of axonal regrowth after SCI.2 Transforming growth factor-β (TGF-β) belongs to a family of cytokines with important functions in inflammation, extracellular matrix formation and scar formation in wound healing.4, 5 In a number of CNS pathologies of experimental animals and humans (including inflammatory disorders, degenerative diseases and traumatic injuries) TGF-β1 and TGF-β2 are rapidly and abundantly expressed.6, 7, 8, 9 In experimental SCI, TGF-β isoforms were shown not only to be involved in the early inflammatory response but also to play a central role in the formation of the scar at the lesion site.8, 10 However, the extent to which experimental SCI induced changes of TGF-β expression reflect the events taking place following human SCI remains unknown. Thus, the present investigation was undertaken to determine the spatio-temporal pattern of TGF-β1 and TGF-β2 expression in samples of post-mortem human spinal cord, taken from patients who died at a range of survival times following severe traumatic SCI. Post mortem, the spinal cords and brains were removed from 4 control patients who had not suffered from any neurological disease and from 14 patients who died at a range of time points after severe traumatic SCI (for details see Table 1). None of the control patients received any immunosuppressive/immunomodulatory medication for at least 3 months before death. Patients with traumatic SCI had been diagnosed as having ‘complete’ injuries and presented with paraplegia or tetraplegia. The study was approved by the Aachen University Ethics Committee. The spinal columns were removed at autopsy, approximately 15–48 h after death. Following incision of the dura mater, the spinal cord was fixed in 4% buffered formaldehyde for at least 2 weeks. Thereafter, blocks of approximately 1 cm thickness were prepared from the lesion epicentre, the intermediate zone, the peri-lesional area and from cervical, thoracic and lumbar levels and were embedded in paraffin wax. For a semiquantitative description of the amount of TGF-β1 and TGF-β2 immunoreactive cells at the various survival times, an arbitrary rating scale for the number of labelled cells was chosen, ranging from 0 to ++++ with 0 indicating no immunopositive cell and ++++ indicating the presence of large numbers of labelled cells. In contrast to the rapid increase in TGF-β1 immunoreactivity, elevated TGF-β2 immunoreactivity was only detected at 24 days after traumatic injury. At this survival time, many cells demonstrated immunoreactivity for TGF-β2, both at the lesion epicentre and in the intermediate zone (Figure 2c). The large, rounded morphology of these cells and double staining for CD68 confirmed the macrophage/microglial identity of the TGF-β2-positive cells (Figures 3f and g). The upregulation of TGF-β2 in the intermediate zone coincided with the appearance of a dense GFAP-positive reactive gliosis/astrocytic scar formation (Figure 2d). By 4 and 10 months after SCI, microglia/macrophages remained TGF-β1 positive at the lesion epicentre and in the intermediate zone, but the number of immunoreactive cells was strongly reduced compared to earlier time points (Figure 2f). Few microglia/macrophages were TGF-β2 immunoreactive at this time. In the intermediate zone, some reactive astrocytes also demonstrated TGF-β1 and TGF-β2 immunoreactivity (mainly at the border of the lesion site, Figures 2e, g, 3h and i) with only occasional cells being detectable by 10 months. Most neurons retained moderate TGF-β1 immunoreactivity up to 10 months post injury, but no nuclear staining could be detected (Figure 2e). In all patients with a survival time of 4 months and longer, the intermediate zone of the lesion site was filled with an irregular, GFAP-positive astrocytic scar (Figure 2h). In cases with survival times ranging from 1 to 30 years, no TGF-β1 or TGF-β2 immunoreactivity could be detected at the lesion epicentre. In the intermediate zone, TGF-β1 immunoreactivity was restricted to occasional motoneurons and blood vessel walls (Figure 2i) while TGF-β2 immunoreactivity was found in occasional, intravascular monocytes (Figure 2j). Inflammation in the CNS, in response to injury, is of major importance but remains only partially understood. Both beneficial and detrimental components of the inflammatory cascade have been identified (for example, Hausmann1). In contrast to studies using experimental animals, there is relatively little correlative information about cytokine expression in human tissues following SCI or insult-induced Wallerian degeneration.11 TGF-β1 and TGF-β2 have previously been shown to be upregulated following experimental spinal cord lesions and to play important roles in the early inflammatory response, in particular being involved in the glial scar formation at and around the lesion site. Since correlative data in human traumatic SCI are lacking, the present immunohistochemical investigation was undertaken to identify the spatio-temporal expression pattern of TGF-β1 and TGF-β2 in both normal and lesioned human spinal cord. The detection of TGF-β1 immunoreactivity that was associated with the endothelium of blood vessel walls probably reflects attachment of the cytokine to extracellular matrix proteins and might play a role in the retention, delivery and clearance of the cytokine.17, 18 The lesion-induced increase of TGF-β1 may exert its pro-inflammatory effects via direct (acting as a potent chemo-attractant) and indirect means (inducing adhesion molecules as well as the degradation of the endothelial basement membrane).4 Furthermore, TGF-β1 stimulates monocytes as well as astrocytes to express a variety of pro-inflammatory cytokines and induces Fcγ receptors on the mononuclear cell surface.4 The anti-inflammatory effects of TGF-β1 are exerted via a number of mechanisms including the modulation of cytokine and chemokine expression, the inhibition of hydrogen peroxide production and the inhibition of the inducible nitric oxide synthase. Furthermore, the anti-inflammatory effect is brought about via indirect means; rendering cells unresponsive to pro-inflammatory cytokines.4 This bi-directional role of TGF-β1 in inflammation results from more pro-inflammatory effects on resting/non-activated cells in the initial phase and more anti-inflammatory effects on activated cells in the later inflammatory cascade. In both experimental animals and humans the prolonged upregulation of TGF-β1 after SCI is in contrast to other cytokines such as interleukin-1β and tumour necrosis factor-α which demonstrate an early but short-lived induction of 1–3 days after trauma.11, 19 The reason for this difference might be that on top of an important function in the early inflammatory cascade, as shared with interleukin-1β and tumour necrosis factor-α, TGF-β1 is also involved in scar formation as previously shown in several experimental studies.8, 10, 19 Lagord et al.8 proposed that TGF-β1 is mainly involved in the inflammatory reactions while TGF-β2 is involved in scar formation following crush injuries to the rat spinal cord. In their study, astroglial scarring and concomitantly TGF-β2 upregulation was detected from around 5 to 28 days after injury. In the present investigation in post-mortem human tissue, the expression pattern of TGF-β2 also correlated well with the formation of the astrocytic scar at the lesion site. The first indication of astrocytic scar formation, 24 days after injury, coincided with the upregulation of TGF-β2 in macrophages at the lesion site. Raised levels of TGF-β2 could be detected for up to a 1-year after injury, by which time, scar formation should have been completed. This expression pattern of TGF-β2 after human SCI is delayed and prolonged compared to animal data but correlates to the delayed formation of astroglial scarring in humans,20 starting approximately 24 days after injury (at which time scarring was nearly complete in the rats8). The reason for delayed scar formation following human SCI is presently not clear but the present results suggest that the lack of an early TGF-β2 upregulation might be involved in this difference. The confirmation of the spatio-temporal upregulation of TGF-β1 and TGF-β2 in human SCI highlights their potential as possible targets for therapeutic interventions aimed at promoting CNS axon regeneration. In experimental studies, the treatment of SCI injured animals with neutralizing antibodies to TGF-β1 and TGF-β2 has demonstrated a reduction in scar formation and a concomitant decrease of associated growth-inhibitory proteins such as chondroitin sulphate proteoglycans (CSPG).21 However, other groups have reported that this approach did not lead to enhanced regeneration of lesioned nerve fibre tracts22 and suggested that the neutralization of TGF-β alone may not be sufficient for promoting functional repair. We thank S Lecouturier for excellent technical assistance. This work was supported by a grant from the Deutsche Stiftung Querschnittslähmung (DSQ).
更多查看译文
关键词
SC, paraplegia, surgery, neurology, spinal cord injury, incontinence
AI 理解论文
溯源树
样例
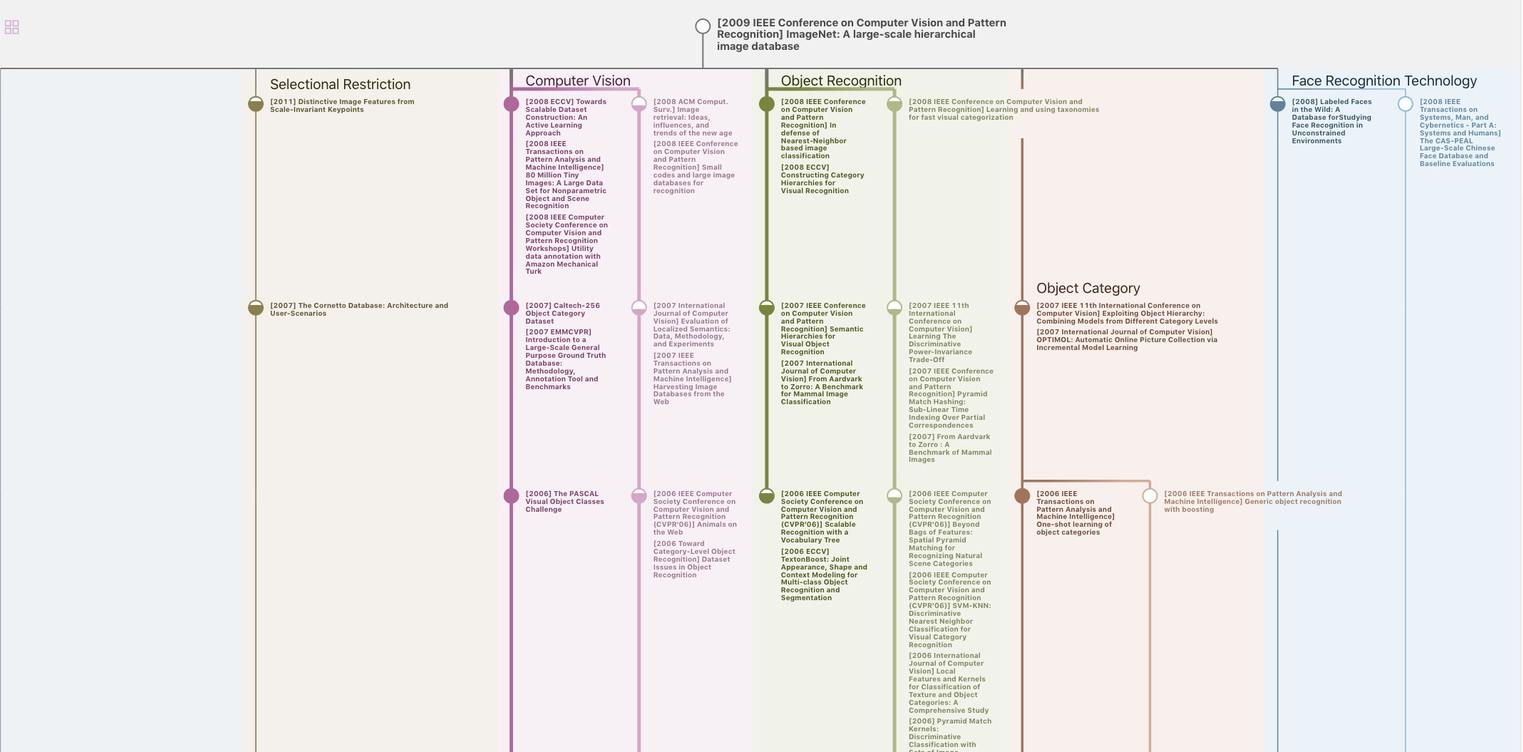
生成溯源树,研究论文发展脉络
Chat Paper
正在生成论文摘要