Molecular biology: DNA replication reconstructed.
NATURE(2015)
摘要
On page 431 of this issue, Yeeles et al.1 report the long-awaited in vitro reconstitution of the initiation of DNA replication using 16 purified proteins from the budding yeast Saccharomyces cerevisiae. This study defines, for the first time, the minimum set of proteins required to initiate DNA replication in eukaryotes (organisms that include plants, animals and fungi). The authors also use their system to examine the regulatory mechanism that limits initiation to just once per cell cycle at each initiation site, confirming and adding key details to what was previously known. Genome duplication must occur before cell division, so that both progeny cells inherit a complete copy of the genetic material. DNA replication is initiated at particular chromosomal sites called origins, after the binding and recruitment of several initiation proteins. In eukaryotes, replication initiation occurs at hundreds to thousands of origins distributed along multiple chromosomes, with one origin for every 40 kilobases of DNA in yeast, and greater intervals in mammals. The activation (firing) of these origins must be strictly regulated so that it occurs only during the DNA-synthesis period (S phase) of each cell-division cycle. Also, each origin must be activated once per cell cycle at most, to avoid potentially lethal over-replication events. Yeeles and colleagues' findings represent a major technical feat that will allow eukaryotic initiation to be studied in detail. The authors' work on in vitro reconstitution would not have been possible without the considerable genetic and biochemical work of the past 30 years — particularly in budding yeast, for which the numerous proteins required for replication initiation have been identified and the complex regulatory processes described2, 3, 4. Not surprisingly, this core replication machinery has been conserved throughout evolution, from yeast to mammals. The initiation of DNA replication can be separated into two distinct and mutually exclusive steps (Fig. 1). In the first step, which occurs in the G1 phase of the cell cycle, an inactive form of a DNA-helicase enzyme complex (MCM) is loaded at all replication origins throughout the genome; DNA helicases separate and unwind the double-stranded DNA helix into two single strands. Strand separation is necessary for the DNA to serve as the template for its own replication, which occurs at protein–DNA structures called replication forks — the points at which the double-stranded DNA is unwound into two single strands. In the second step of initiation, the loaded DNA helicase is activated by the recruitment of further protein components. Helicase activation occurs locally at each active origin just before and during S phase. Initiation proteins also recruit DNA primase and DNA polymerases, enzymes required to synthesize two daughter strands on the basis of the template sequence of the parental strands. To prevent any single origin from firing more than once per cell cycle, the helicase must be loaded at the beginning of the cell cycle (G1 phase), the only period in which the activity of the cyclin-dependent kinase (CDK) enzyme is low. CDK belongs to the protein-kinase family of enzymes, which covalently modify a protein or small molecule by adding a phosphate group, thereby changing the molecule's biological properties. High CDK levels prevent further helicase loading, but are required for helicase activation. CDK activity thus acts as a switch between the two replication steps. A second protein kinase, DDK, is also required for helicase activation and accumulates towards the end of G1 phase. This intricate coupling of helicase loading and activation to protein-kinase activity is necessary to prevent re-replication of the genome5. The activation of origins outside S phase, or more than once per cell cycle, would generate amplified DNA regions that could break when chromosomes are segregated during mitosis; such breaking would probably be lethal. Yeeles and co-workers' study defines the minimum set of protein substrates for CDK and DDK that are required for initiation in vitro, and confirms previous work5. CDK and DDK phosphorylate many amino-acid residues in their substrates, so researchers can now use the authors' in vitro system to map which phosphorylation events are essential and how these modifications affect protein–protein interactions and biological activities. The MCM helicase, which is composed of six subunits, is loaded around double-stranded DNA as a dodecamer consisting of two identical hexamers in a 'head-to-head' arrangement6. How this occurs is still an open question, because the only identified intermediates7 for this process have a single hexamer engaged with double-stranded DNA. It will be important to determine whether the two hexamers are loaded by two helicase loaders, sequentially by one helicase loader, or perhaps as an intact dodecamer8. This work also opens up many other areas of investigation, such as how the helicase is activated, and how polymerases are recruited and coupled to the helicase at the replication fork. Helicase activation requires CDK, DDK and two other proteins to form a complex called the CMG helicase9, 10. However, Yeeles and colleagues find that CMG cannot unwind origin DNA effectively by itself — another protein (Mcm10) is also required. Investigating the interactions between the helicase subunits, how each contributes to the initial unwinding event and how the initial dodecamer is split into two hexameric units, are exciting research opportunities that are now clearly possible. Yeeles et al. observe that the DNA polymerase required for leading-strand synthesis is recruited during the helicase activation step. However, their in vitro system currently lacks other components needed for chromosomal replication. These include: the DNA polymerase that makes the lagging strand; the sliding 'clamp' that tethers DNA polymerases to DNA; the clamp loader; a triad of proteins called the fork-protection complex; and the proteins required for the final processing steps (maturation) of the replication products. Much work is therefore still required to reconstitute the complete replication reaction. If an in vitro system could be devised that incorporates a synthesis reaction including both leading and lagging strands, one could imagine reconstituting the assembly of chromatin — the complex of histone proteins and DNA in the cell nucleus — behind each fork, as occurs in cells. Chromatin, rather than naked DNA, is the true template for DNA replication in the cell. Finally, it will be interesting to see whether the authors' system could eventually be used to investigate mechanisms for maintaining fork stability and for replication-coupled DNA repair. These crucial processes are clearly involved in maintaining genome integrity, and are of particular interest because they are often lost in cancerous cells. Download references
更多查看译文
关键词
Molecular biology, Biochemistry
AI 理解论文
溯源树
样例
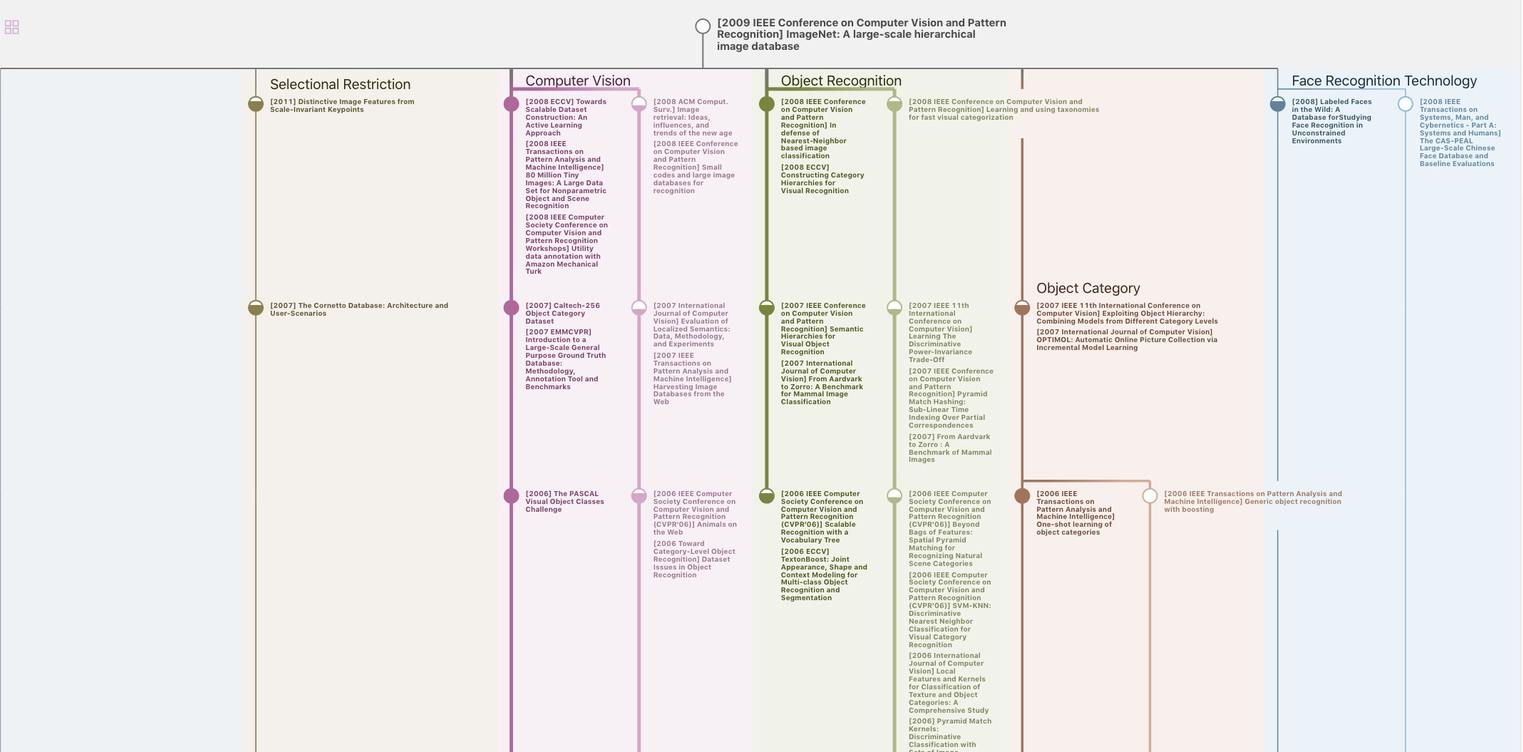
生成溯源树,研究论文发展脉络
Chat Paper
正在生成论文摘要