Phase I and pharmacodynamic study of high-dose NGR|[ndash]|hTNF in patients with refractory solid tumours
British Journal of Cancer(2013)
摘要
Tumour necrosis factor (TNF)-alpha is a powerful antitumour cytokine originally identified (Carswell et al, 1975) for its ability to induce in experimental cancers massive haemorrhagic necrosis, which is mainly mediated by apoptosis of cancer endothelial cells. The apoptotic pathway is triggered by TNF binding with the receptor TNF-R1. Conversely, the receptor TNF-R2 lacks a death domain and likely acts as a modulator of TNF-R1 actions. Both receptors are also shed as soluble proteins, thus competing with cell-surface receptors for free ligand (Balkwill, 2009). Early clinical trials with TNF given systemically were, however, associated to severe toxicities, with maximum-tolerated dose (MTD) being significantly lower than estimated effective dose (Lejeune et al, 2006). Lately, through isolated limb perfusion it was possible to deliver loco-regionally doses of TNF 10-fold higher than MTD. Of note, TNF in combination with chemotherapy induced high response rates in several tumours, with an acceptable toxicity profile (Fraker et al, 1995). In order to increase the TNF therapeutic index, a ligand-directed vascular-targeting approach was exploited. For this purpose, NGR–hTNF was prepared by conjugating the N-terminus of TNF with the C-terminus of the tumour-homing peptide NGR (asparagine–glycine–arginine) that selectively recognises an aminopeptidase N (CD13) isoform overexpressed by angiogenic tumour vessels (Arap et al, 1998; Curnis et al, 2000). Recently, CD13 was found to be crucial for the pathological development of newly formed blood vessels from preexisting ones in CD13-null mice (Rangel et al, 2007). Compared with untargeted TNF, NGR–TNF induced a significantly increased antitumour activity in preclinical models and, more interestingly, displayed a biphasic (U-shaped) dose–response curve with activity noted either at very low (0.1 ng) or at high doses (>1000 ng) (Curnis et al, 2002). A previously reported phase I study (van Laarhoven et al, 2010) testing doses ranging from 0.2 to 60 μg m−2 established the MTD of NGR–hTNF at 45 μg m−2 given intravenously as 1-h infusion once every 3 weeks, with dose-limiting toxicities (DLTs) encountered at the dose of 60 μg m−2 being grade 3 infusion-related reactions (IRRs). Common related toxicities were transient grade 1 to 2 chills (58% of patients) and fever (56%). Shedding of soluble TNF receptors was not detected at the lowest doses tested (0.8 μg m−2), while thereafter increased proportionally with dose up to a plateau observed at 25 μg m−2. No objective responses were detected, but of six patients with durable stable diseases, five were treated with low doses. An additional trial exploring the low-dose range from 0.2 to 1.6 μg m−2 selected 0.8 μg m−2 as optimal biological low dose, based on dynamic imaging changes and sTNF-Rs shedding kinetics (Gregorc et al, 2010). In this study, the antivascular effects of NGR–hTNF were confirmed by dynamic contrast-enhanced magnetic resonance imaging (DCE-MRI), with 75% of patients having decreased values of the volume transfer constant, Ktrans. Considering the preclinical activity noted only at very high doses and the temporal relationship between DLTs onset and drug dosing, we decided to explore higher doses of NGR–hTNF using a more protracted infusion length of 2 h and a mild premedication with paracetamol. The present study primarily aimed at determining the optimal biological high dose of NGR–hTNF, given at doses higher than the previously established MTD, by evaluating both safety, in terms of MTD, and antivascular effects, in terms of changes in dynamic imaging. Secondary aims included pharmacokinetics, pharmacodynamics, and preliminary activity. Adverse events (AEs) were graded according to Common Terminology Criteria for Adverse Events (version 3.0). Dose-limiting toxicities applicable to the study were defined as any grade 3 or 4 toxicity clearly related to NGR–hTNF. Exceptions were considered nausea, vomiting, chills, and fever that could be rapidly controlled with appropriate treatments. Four patients were to be enroled to each DL. After enrolment of the first two patients, new patient inclusion was blocked until the safety of the first two patients to the first cycle was fully documented in order to verify that no DLT had occurred. Then, subsequent two patients were allowed for registration at that particular DL. If one or less of the four patients experienced a DLT during the first cycle, an additional four patients were entered at the next higher DL with dose escalation continuing until DLT was observed. If two or more of the four patients experienced DLT during the first cycle, the prior lower DL would be declared as MTD. The MTD was not reached and no DLTs were observed. Overall, NGR–hTNF was well tolerated without apparent differences in either frequency or intensity of AEs by dose. Most common AEs over all cycles, regardless of drug relationship, were pyrexia and chills (Table 1), with grade 3 and 4 AEs reported by 12 (25%) and 5 (10%) patients, respectively. No patient experienced grade 3–4 drug-related AEs, while grade 1–2 IRRs included chills (58%), vomiting (44%), and hypotension (25%). During the first cycle, chills was noted in 24 patients (50%). The antivascular effects of NGR–hTNF were evaluated in 37 patients who had at least one post-baseline DCE-MRI assessment. As shown in Table 2, both Ktrans and IAUGC were markedly reduced after first cycle with an apparent dose-dependent effect, as the changes in Ktrans correlated with both Cmax (r=−0.35; P=0.03) and AUC (r=−0.31; P=0.06) of NGR–hTNF. Overall, 28 patients (76%; 95% CI, 59–88%) had reduced values of Ktrans and IAUGC at some point on treatment, and 16 patients (43%; 95% CI, 27–60%) experienced decreases in Ktrans from baseline >50% (Figure 2). In particular, patients receiving DLs 200 μg m−2 had significantly greater reductions in Ktrans than patients receiving DLs <200 μg m−2 (median, −52% and −24%, respectively; P<0.0001) (Figure 3A). Moreover, for higher baseline Ktrans values, greater decreases were noted. Indeed, the absolute changes in Ktrans were inversely correlated with pre-treatment values (r=−0.58; P=0.0002), particularly in the subset of 28 patients who had decreased values (r=−0.85; P<0.0001) (Figure 3B). There was no correlation between pre-treatment tumour size and either baseline or post-treatment Ktrans values. Decreases in Ktrans were reported in 16/22 patients with colon cancer, in 5/6 with mesothelioma, in 2/4 with sarcomas, in 3/3 with liver cancer, and in one patient each with gastric cancer and PNET. Drug doses are established in phase I studies, but infusion length or administration frequency are commonly determined empirically based on available preclinical data. Then, subsequent observations can either reinforce or modify these decisions. However, often dosing schedules are based on other considerations, such as volume necessary for administration or, most importantly, safety. The MTD of NGR–hTNF was established at 45 μg m−2 as 1-h intravenous infusion in a previous study testing a dose interval from 0.2 to 60 μg m−2, with DLTs consisting of grade 3 IRRs (van Laarhoven et al, 2010). In that study, the infusion length was not further prolonged because it was assumed that, given a dose-proportional increase of NGR–hTNF exposure and a plateau of sTNF-Rs shedding, the excess of free drug unbound to circulating receptors at higher doses would be responsible for DLTs. Therefore, further prolongation of the infusion time was not expected to alter toxicity. Though severe reactions are rare, mild-to-moderate IRRs occur frequently with many compounds and tend to resolve after brief infusion interruption and supportive care administration (Lenz, 2007). Furthermore, most patients experiencing mild-to-moderate IRRs during first exposure tend to tolerate drug rechallenge with slower infusion rate and premedication. Therefore, in the present study a more prolonged infusion time empirically set at 2 h was explored, so that if IRRs were detected, the infusion could be interrupted with a relatively small amount of drug having been delivered. Moreover, considering that the vast majority of these IRRs consisted of chills, a mild premedication with paracetamol was mandated starting from first cycle. The present study has clearly demonstrated that NGR–hTNF can be safely escalated at doses up to seven times higher than the previous MTD by slowing the infusion rate at 2 h and using a mild premedication with paracetamol. The use of high doses did not change the toxicity pattern reported for single-agent NGR–hTNF, mainly consisting of mild-to-moderate and dose-unrelated chills. Similarly, the cardiovascular toxicities described for vascular-targeting agents (including acute coronary and thrombophlebitic syndromes; alterations in blood pressure, heart rate, and ventricular conduction) (van Heeckeren et al, 2006) did not appear in this study, likely because of targeted delivery of this compound. As expected using a targeted agent and despite the wide dose interval tested, the present trial did not formally define a new MTD. However, the present study primarily aimed at determining the optimal biological high dose of NGR–hTNF by evaluating both safety and antivascular effects by dynamic imaging. Furthermore, it is now widely agreed that the toxicity spectrum of targeted agents differs profoundly from the one of cytotoxic agents and, given their cytostatic nature and lack of dose–effect relationship, increasing drug dose to MTD may be unnecessary for drug effect (Ellis and Hicklin, 2008). Notwithstanding the fact that the selection of an optimal biological dose is highly challenging, it is worthy to note that the low dose of 0.8 μg m−2 was previously selected based on more pronounced antivascular effects and no shedding of soluble TNF receptors noted at this dose level (Gregorc et al, 2010). These circulating receptors might compete with the cell-surface receptors for free TNF, thus blocking its bioavailability and activity, with the amount and speed of this shedding being linearly correlated with serum TNF level (Aderka et al, 1998). The plateau in sTNF-Rs shedding kinetics observed previously (van Laarhoven et al, 2010) and confirmed also in the present study is consistent with a biphasic dose–response curve and suggests that high doses of NGR–hTNF can overcome this counterregulatory mechanism. DCE-MRI scans were done at baseline and 2 h after NGR–hTNF dosing to measure the biological effects on tumour vascularity. Shortly after a single dose, NGR–hTNF markedly decreased Ktrans, and doses higher than 200 μg m−2 appeared to be the most effective, with median reductions of >50%. It is important to emphasise that Ktrans is a mixed measure of tumour vascularity and permeability. Thus, Ktrans measurements could be influenced by several factors, including tumour types and analysis models. In the present study, there was no indication that any particular tumour type was overrepresented among tumours with either more dramatic decreases or higher baseline Ktrans values. Interestingly, a correlation between baseline values and post-treatment changes in Ktrans was noted, thus suggesting increased antivascular effects in tumours with extensive abnormal vasculature. The fact that these decreases in tumour permeability and blood flow occur so rapidly can be interpreted either as normalisation of tumour vasculature owing to effects on integrity (permeability) and function (vessel perfusion) of vascular bed, or vascular damage. Both mechanisms are expected, however, to improve the synergy with chemotherapy by increasing the intratumoural chemotherapy uptake or sensitising the tumour vascular compartment to cytotoxic effects. Therefore, both mode of action of NGR–hTNF and nonoverlapping toxicity profile with cytotoxic agents could facilitate the combination with chemotherapy. Consistently, NGR–hTNF has been reported to synergise with several chemotherapeutic agents (Sacchi et al, 2006). Even though a short median PFS of 1.7 months was registered for the overall study population, this figure seems to be substantially in line with median PFS of 2.5 months reported in literature for heavily pretreated patients enroled in phase I trials (Arkenau et al, 2008). Interestingly, the early post-treatment findings that the lower the Ktrans and sTNF-Rs values, the higher the patient survival rates, deserve further investigations in larger patient population to clarify their role as suitable tools for either prognostication or prediction. In conclusion, this study has shown that NGR–hTNF can be safely given at doses higher than the previous MTD using a mild premedication and a longer infusion time. The observed tolerability profile and biological effects warrant further clinical testing of doses higher than 200 μg m−2 of NGR–hTNF combined with chemotherapy and compared with a low dose of 0.8 μg m−2.
更多查看译文
关键词
nature, nature publishing group, BJC, British Journal Cancer, cancer research, cancers, prescription drugs, breast cancer, medical research laboratory, lung cancer, nature, prostate cancer, skin cancer, leukaemia, colon cancer, ovarian cancers, cervical cancer, liver cancer, cancer treatments, brain cancer, gene therapy, bone marrow, apoptosis, nature magazines, bone marrow transplant, science news articles, cell division, cancer cells, nature journals, oncogene, neoplasia, antioxidants, adipose tissue, science and nature, oncogene journals, tumours, cancer gene therapy, apoptosis pathway, anti cancer drugs, science research papers, anticancer
AI 理解论文
溯源树
样例
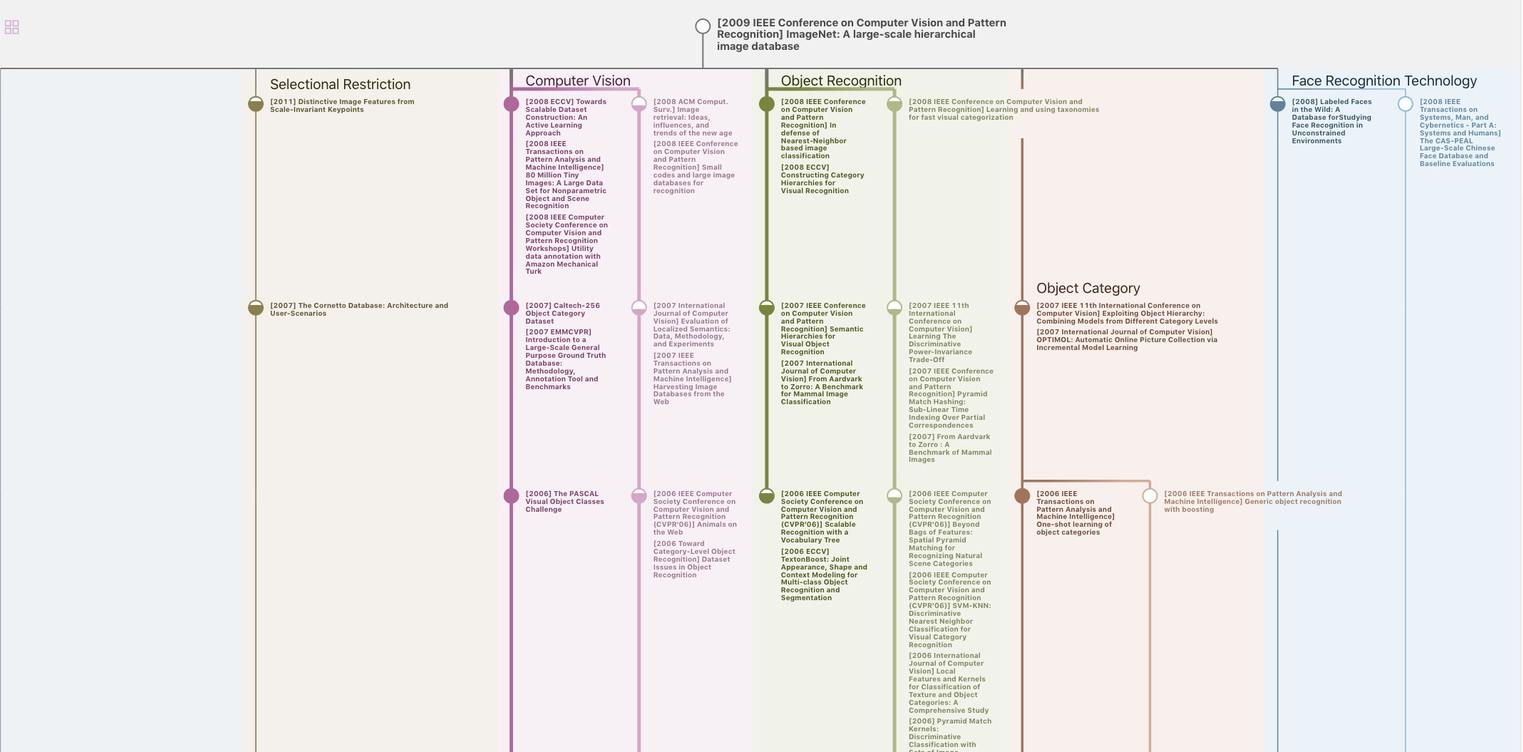
生成溯源树,研究论文发展脉络
Chat Paper
正在生成论文摘要