Analysis of Stent-Graft Design for Aortic Aneurysm Repair Using Computational Fluid Dynamics
JOURNAL OF MEDICAL DEVICES-TRANSACTIONS OF THE ASME(2015)
摘要
Aortic aneurysms have been a significant issue within the vascular surgical field. Two main surgical repair procedures have been developed over time to treat these aneurysms: open repair and endovascular repair. The endovascular method is often preferred, as it reduces the amount of aortic clamp time and physiological insult to the body, effectively reducing the risk of life-threatening complications. The drawback of this endovascular surgical method, however, is that it may not be as durable as an open surgical repair, especially in terms of branch vessel patency.Historically, clinical trials have been the best method to determine patency, life expectancy, and other postoperative conditions based on new bridging stent configurations. Though these trials are the most realistic form of experimental testing, they are not ideal because they use a human body as the testing mechanism. Laboratory experimental tests are not particularly feasible because of the intricate conditions of blood flow within the vascular system.These challenges have led to computational fluid dynamics (CFD) coming to the forefront as a safe, reliable, time-efficient manner of testing new aortic stent-graft designs. CFD can readily analyze different geometrical features side-by-side, including velocity profiles, wall shear stresses, and other important parameters, to see what design conditions are optimal for the highest quality flow through a graft. The overall goal of this study was to provide a robust method for easily analyzing any kind of stent-graft for aneurysm repair using the computational tool of CFD.CFD software was used to analyze a variety of commercially available stent-grafts in order to evaluate their performance comparatively. CFD solves the complicated second-order differential Navier–Stokes equations to simulate the fluid dynamics and flow mechanics throughout a system. The CFD software star-ccm+ version 9.04 by CD-Adapco was used to perform the fluid analysis on 3D models created in PTC CREO 2.0 CAD software.Three stent-grafts chosen for this study can be seen in Fig. 1: a fenestrated [1], branched [2], and chimney graft [3]. The fenestrated graft uses renal bridging stents that force blood to make a 90 deg change in direction to transport to the arteries, whereas the branched stents are 30 deg off of the main body. The chimney graft pulls flow from the main body cross section, requiring little momentum change by the blood.These geometries were idealized so that the distal end of the renal, celiac, and superior mesenteric arteries (SMA) would all have the same relative locations for each graft. The renal arteries in each graft were given a constant diameter of 6 mm, while the celiac artery and SMA were given constant diameters of 8 mm and 7 mm, respectively. To simplify the simulations, the walls of the system were modeled as rigid and nondeforming with a no-slip boundary condition. It was determined that, since the scope of this study is to provide a relative comparison of the basic fluid mechanics of these three grafts, idealized geometries with smooth vessels and walls would be sufficient as long as each graft was given those same characteristics.The simulations were run in transient state in order to take into account the unsteady, pulsatile nature of the blood flow through the aorta. The flow profile was made to follow the trend of a typical cardiac cycle [4]. The outlets were determined to be constant flow-split outlets [4]. For a subject at rest and fasting, it was determined that 21% of the supraceliac aortic flow would go to the celiac, 16% to the SMA, 15% to each renal, and 16.5% to each iliac artery [4].Based on the size and flow rate of the aorta and each artery, the system was determined to have laminar flow, with a blood density of 1050 kg/m3 [4]. Validation simulations were initially run using a low-Reynolds number turbulence solver to see if sharp bifurcations inducing turbulence would have an effect on the modeling. However, the differences were negligible, so the simulations used a laminar flow regime to save computational time. Blood flow is considered to be non-Newtonian in vessels with diameter less than 1 mm. Since the smallest vessel in these simulations is the renal (6 mm), blood was assumed to be Newtonian throughout, having a constant dynamic viscosity of 0.004 Pa s.For each simulation, a polyhedral mesher with minimum surface size of 0.25 mm was used, resulting in a range of cell numbers from 411,122 (fenestrated) to 618,287 (branched), based on the overall volume and complexity of the graft. Using this detailed mesh, it was determined that model convergence would be achieved at residuals of 0.001 for continuity for each timestep.For the scope of this study, time-averaged wall shear stress (TAWSS) and oscillating shear index (OSI) were used to analyze the grafts and their performance at the renal bridging stent. These parameters have historically been used as a basis for predicting the likelihood of a variety of vascular phenomena. TAWSS is defined in the following equation:(1)TAWSS=1T∫0T|τw|dtLow TAWSS (<0.4 Pa) can delay the providing of nutrients and the removal of waste at those locations [5]. However, high TAWSS (>7 Pa) can cause endothelial damage and plaque rupture, which can lead to shear thrombosis [5]. Additionally, high gradients of TAWSS can lead to endothelial tension [5]. OSI is defined in the following equation:(2)OSI=0.5(1-|∫0T→τWdt|∫0T|→τW|dt)OSI indicates oscillations in wall shear stress direction due to recirculation and stagnation. High OSI (>0.3) can lead to erratic endothelial cell growth and atherosclerosis [5].After running the simulations in star-ccm+, the TAWSS throughout the renal branching stent of each graft was plotted, as can be seen in Fig. 2. The fenestrated device had elevated TAWSS near the proximal end of the bridging stent, caused by the sharp acceleration and change in direction of the blood flow. The branched graft had a high degree of TAWSS gradient near the proximal end. The chimney graft had a smooth TAWSS profile.Additionally, the OSI plots were generated for the renal stent of each device, as can be seen in Fig. 3. For the fenestrated graft, there is a spot of relatively high OSI just after the branch off the main body. The conglomeration of these high OSI cells is a concern, increasing the probability of significant atherosclerosis and plaque build-up. For the branched graft, a large concentration of elevated OSI cells exists toward the distal end of the bridging stent. This may be a greater issue than on the fenestrated graft, as this location is closer to where the renal artery wall would begin. The issues of atherosclerosis can become much more profound when occurs within the renal artery. The chimney graft had small areas of relatively high OSI near the bifurcation, but consistently low values through the rest of the stent.Based on the comparisons of these grafts, it was determined that longer, gradual sweeps for the renal bridging stents is preferred, as opposed to short, sharp angles that require significant changes in the direction of the blood flow. Overall, it should be noted that any high TAWSS values were significantly lower than the dangerous level of 7 Pa. However, these grafts were idealized models. TAWSS will significantly increase if there is any sort of plaque build-up or blockage that begins to form. Thus, those relatively high TAWSS values from this idealized case could easily indicate instability that could promote thrombosis. The same can be said for the OSI plots, as elevated OSI values, though not immediately dangerous, can easily become significantly high with any sort of blockage to cause more momentum changes and flow reversals within the bridging stent.This study provides a template for reliable, safe, and time-efficient means of analyzing new stent-graft designs for implementation in any aneurysm using CFD. Using this analysis, grafts can be more easily designed in order to ensure the highest quality of life for the patient years after surgery.
更多查看译文
关键词
computational fluid dynamics,design
AI 理解论文
溯源树
样例
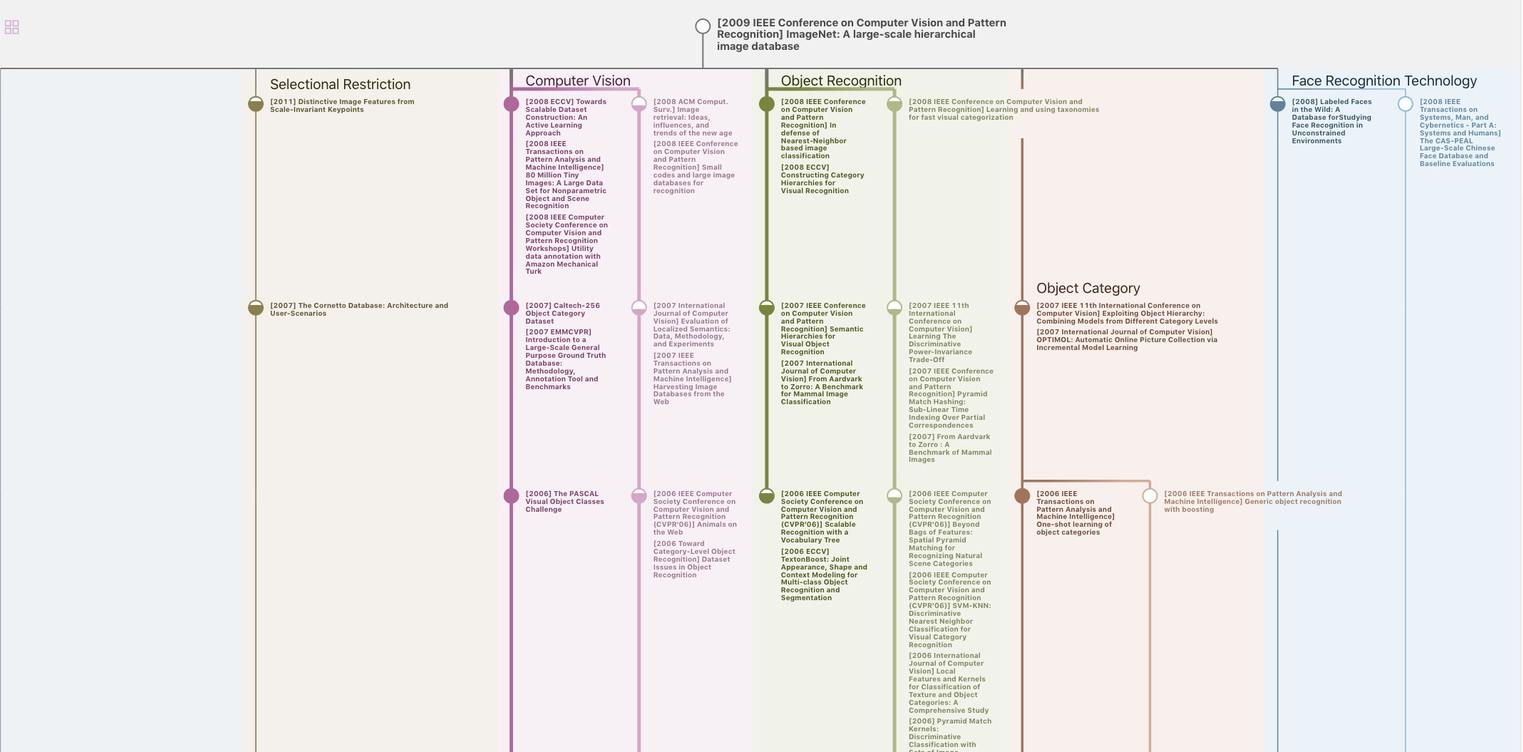
生成溯源树,研究论文发展脉络
Chat Paper
正在生成论文摘要