Zhou Substrate Adaptor DCAF 1 FERM Domain to the E 3 Ubiquitin Ligase Structural Basis of the Binding of Merlin Developmental
semanticscholar(2014)
摘要
The tumor suppressor gene Nf2 product, Merlin, plays vital roles in controlling proper development of organ sizes by specifically binding to a large number of target proteins localized both in cytoplasm and nuclei. The FERM domain of Merlin is chiefly responsible for its binding to target proteins, although the molecular basis governing these interactions are poorly understood due to lack of structural information. Here we report the crystal structure of the Merlin FERM domain in complex with its binding domain derived from the E3 ubiquitin ligase substrate adaptor DCAF1 (also known as VPRBP). Unlike target binding modes found in ERM proteins, the Merlin-FERM binding domain of DCAF1 folds as a β-hairpin and binds to the α1/5-groove of the F3 lobe of Merlin-FERM via extensive hydrophobic interactions. In addition to providing the first structural glimpse of a Merlin-FERM/target complex, the structure of the Merlin/DCAF1 complex is likely to be valuable for understanding Merlin’s interactions with its binding partners other than DCAF1. http://www.jbc.org/cgi/doi/10.1074/jbc.M114.551184 The latest version is at JBC Papers in Press. Published on April 4, 2014 as Manuscript M114.551184 Copyright 2014 by The American Society for Biochemistry and Molecular Biology, Inc. at H ng K ng U niersity of Sence & T ecology on M ay 4, 2014 hp://w w w .jb.org/ D ow nladed from Structure of the Merlin FERM/DCAF1 complex 2 Cell-to-cell contact triggers inhibition signals for cell growth, which is crucial for living systems to maintain proper organ sizes by balancing the rate of cell proliferation and apoptosis. Disruption of the contact inhibition gives rise to cell overgrowth and often induces tumor formation (1). The tumor suppressor gene Nf2, which encodes the protein 4.1, Ezrin, Radixin, Moesin (FERM) domain-containing protein Merlin, is an important determinant in contact-mediated cell growth inhibition (2). Consistent with its critical role in growth inhibition, loss-of-function mutations of Merlin are known to cause a series of tumor formations, including the familial cancer syndrome neurofibromatosis type 2 and several other carcinomas (3–6). Mechanistically, Merlin is known for its central roles in the Hippo pathway in controlling organ sizes (7, 8). Recent investigation indicated that Merlin is also involved in a distinct cell growth regulation mechanism by accumulating in nucleus and inhibiting the activity of nuclear E3 ubiquitin ligase via binding to its substrate adaptor DCAF1 (also known as viral protein R-binding protein, VPRBP) (9). The interaction between Merlin and DCAF1 is mediated by the FERM domain of Merlin (Merlin-FERM) and the C-terminal tail of DCAF1 (DCAF1-CT) (9). However, the molecular basis for the FERM-mediated Merlin/DCAF1 interaction remains unknown. FERM domain is a well-known protein binding module which is composed of three subunits, called F1, F2 and F3 lobes (10). Numerous FERM domain-containing proteins have been identified and classified into different subfamilies (10). As one of the best studied subfamily of FERM superfamily, Ezrin, Radixin, and Moesin (ERM) are the most closely related ones to Merlin. Similar to ERM proteins, Merlin has ~600 residues in length and contains the N-terminal FERM domain, a central helical region, and a C-terminal regulatory domain (CTD) (Fig. 1A). Despite of the high sequence similarity with ERM proteins, especially their FERM domains (sequence identity of ~60%), Merlin was shown to have distinct tissue localization and functions (11). This functional specificity indicates that the target binding mechanism of Merlin is different from ERM proteins. Extensive studies have implicated a number of potential Merlin targets (8, 9, 12, 13), most of which (including DCAF1) were found to interact with its FERM domain. Structural studies confirmed that Merlin-FERM share high structural similarity with the FERM domains of ERM proteins (14, 15). However, due to lacking of target-bound structures of Merlin-FERM, the mechanisms underlying target recognitions of Merlin were modelled largely based on the structures of ERM proteins (especially Radixin) in complex with their targets. Here, we show that Merlin-FERM directly binds to a short fragment of DCAF1 located at the extreme C-terminal end (termed FERM binding domain or FBD, Fig. 1A). We determined the crystal structure of Merlin-FERM/DCAF1-FBD complex at the 2.6 Å resolution. In addition to uncovering the molecular basis governing the specific Merlin-FERM/DCAF1 interaction, the complex structure also reveals a distinct FERM-mediated targeting mechanism in general. Unlike target binding modes found in ERM proteins, DCAF1-FBD folds as a β-hairpin to augment the β-sheet of the F3 lobe of Merlin-FERM via extensive hydrophobic interactions. Additionally, the highly conserved, negatively charged DCAF1 β-hairpin loop was found to contribute to the DCAF1/Merlin interaction, likely via binding to a positively charged cleft between the F1 and F3 lobes of Merlin-FERM. EXPERIMENTAL PROCEDURES Protein expression and purification – The Merlin constructs (Merlin-FL, residues 1-596; Merlin-FERM, residues 1-313), and the DCAF1 at H ng K ng U niersity of Sence & T ecology on M ay 4, 2014 hp://w w w .jb.org/ D ow nladed from Structure of the Merlin FERM/DCAF1 complex 3 constructs (DCAF1-CT, residues 1417-1507; DCAF1-FBD, residues 1478-1507) were amplified by PCR using the mouse and human cDNA libraries as the template, respectively, and individually cloned into the modified pET32a vector. Various mutants were created using standard two-step PCR-based methods and confirmed by DNA sequencing. Recombinant proteins with N-terminal Trxand His6-tagged were transformed to E. coli BL21(DE3) cells, cultured at 37 °C to OD ~ 0.6 and induced with 0.2 mM IPTG at 16 °C overnight. The expressed proteins were purified by a Ni-NTA agarose affinity chromatography followed by a size-exclusion chromatography. During purification, all protein samples were detected and analyzed by SDS-PAGE coupled with Coomassie blue staining. Isothermal titration calorimetry (ITC) assay – ITC was carried out on a MicroCal VP-ITC at 25°C. All proteins were dissolved in a buffer containing 50 mM Tris pH7.5, 250 mM NaCl, 1 mM DTT, and 1 mM EDTA. The titration processes were performed by injecting 5-10 μL aliquots of protein samples in syringe (concentration of 100 μM) into protein samples in cell (concentration of 10 μM) with 27 times and at time intervals of 120s to ensure the titration peak returned to the baseline. The data were analyzed using the Origin 7.0 and fitted by the one-site binding model. Crystallization – For crystallization, the tagged proteins were treated by a small amount of HRV 3C protease at 4°C overnight to cleave the fusion tags and further purified by a size-exclusion chromatography. Crystals of the Merlin-FERM/DCAF1-FBD complex were obtained by hanging drop vapor diffusion method at 16°C within 5 days. To set up a hanging drop, 1 μL of concentrated protein mixture (~20 mg/mL) at 1:1 stoichiometric ratio was mixed with 1 μL of crystallization solution with 20% Isopropanol and 5% PEG8000, pH 8.0. Before diffraction experiments, crystals were soaked in crystallization solution containing 30% glycerol for cryoprotection. The diffraction data were collected at Shanghai Synchrotron Radiation Facility and were processed and scaled using HKL2000 (16) (Table 1). Structure determination – The initial phase was determined by molecular replacement using the apo form of Merlin-FERM (PDB code: 1ISN) as the searching model. The model was refined in Phenix (17) against the 2.6 Å dataset. The DCAF1-FBD peptide was built subsequently in COOT (18). In the final stage, an additional TLS refinement was performed in Phenix. The final model was further validated by using MolProbity (19). The refinement statistics are listed in Table 1. The structural model of DCAF1-FBD was well assigned except for the connecting loop for the β-hairpin structure. All structure figures were prepared using PyMOL (http://pymol.sourceforge.net/). The sequence alignments were prepared and presented using ClustalW (20) and ESPript (21), respectively. RESULTS The FERM domain of Merlin specifically binds to a short, C-terminal tail fragment of DCAF1 – Merlin-FERM was shown to interact with the DCAF1 C-terminal region recently (9). We first tried to verify this interaction using purified recombinant proteins. Quantitative binding assays showed that the C-terminal region of DCAF1 (DCAF1-CT, residues 1417-1507) binds to Merlin-FERM with a dissociation constant (Kd) of ~3 μM (Fig. 1B). Further boundary mapping revealed that the N-terminal part of DCAF1-CT (residues 1417-1477) showed no detectable binding to Merlin-FERM (Data not shown), and the remaining C-terminal 30 residues (residues 1478-1507) displayed the same binding affinity as the entire DCAF1-CT does (Fig. 1C), indicating that the last 30 residues in DCAF1 contains the complete FERM binding domain (DCAF1-FBD). The binding of Merlin-FERM to DCAF1-FBD is mainly driven at H ng K ng U niersity of Sence & T ecology on M ay 4, 2014 hp://w w w .jb.org/ D ow nladed from Structure of the Merlin FERM/DCAF1 complex 4 by enthalpy (Fig. 1B&C). Interestingly, although sharing a high amino acid sequence identity with Merlin, the FERM domain of Moesin had no detectable binding to DCAF1-FBD (Fig. 1D), indicating that the FERM domain of Merlin encodes its intrinsic target binding specificities. A Merlin-FERM chimera (termed as Merlin-FERM), in which the F3 lobe was replaced by the corresponding F3 lobe of Moesin, failed to bind DCAF1-FBD (Fig. 1E), indicating that the F3 lobe is chiefly responsible for Merlin-FERM to bind to DCAF1. Overall Structure of the Merlin-FERM/DCAF1-FBD complex – To understand the molecular basis governing the Merlin/DCAF1 interaction, we determined the crystal structure of Merlin-FERM in complex with DCAF1-FBD at 2.6 Å resolution using t
更多查看译文
AI 理解论文
溯源树
样例
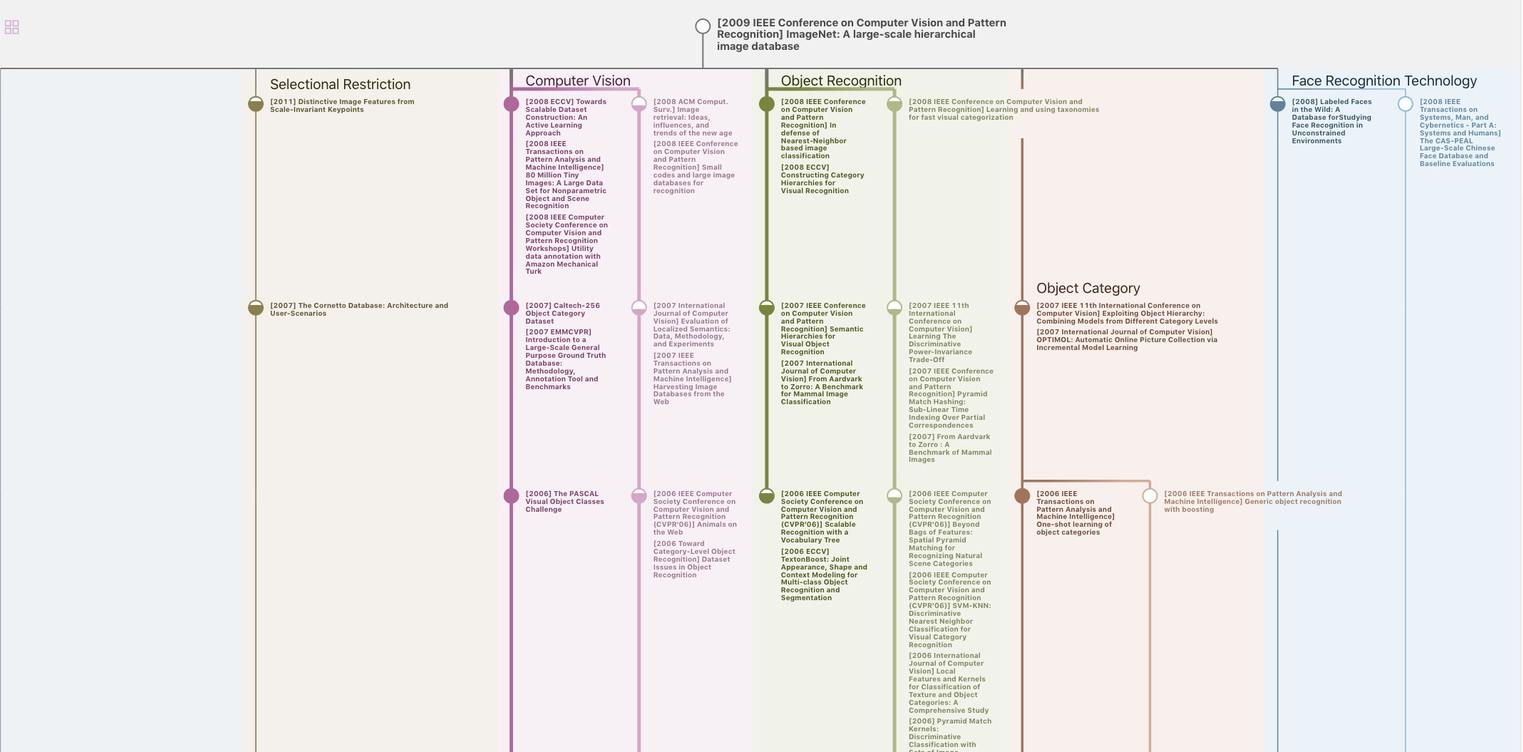
生成溯源树,研究论文发展脉络
Chat Paper
正在生成论文摘要