Thesis: A Numerical Study of Continuous Data Assimilation for the 2D Navier– Stokes Equations Using Nodal Points
semanticscholar(2013)
摘要
s of Publications Finite Fractal Dimension and Hölder–Lipschitz Parametrization C. Foias and E. Olson Abstract. In this paper we first extend the Hölder-Mañé Theorem [BenArtzi–Eden–Foias–Nicolaenko 1993] from a finite dimensional space to the general Hilbert space setting. Namely, if H is a real Hilbert space and X ⊆ H has fractal dimension less than m/2, then for any orthogonal projection P of rank m and δ ∈ (0, 1) there is an orthogonal projection P̃ such that ‖P − P̃‖ < δ and P̃ |X has Hölder inverse. Moreover, for any metric space M of finite fractal dimension less than m/2 there exists a Lipschitz function g:H → R with Hölder inverse on its image. In this paper we first extend the Hölder-Mañé Theorem [BenArtzi–Eden–Foias–Nicolaenko 1993] from a finite dimensional space to the general Hilbert space setting. Namely, if H is a real Hilbert space and X ⊆ H has fractal dimension less than m/2, then for any orthogonal projection P of rank m and δ ∈ (0, 1) there is an orthogonal projection P̃ such that ‖P − P̃‖ < δ and P̃ |X has Hölder inverse. Moreover, for any metric space M of finite fractal dimension less than m/2 there exists a Lipschitz function g:H → R with Hölder inverse on its image. The Camassa–Holm Equations as a Closure Model for Turbulent Channel Flow Shiyi Chen, Ciprian Foias, Darryl D. Holm, Eric Olson, Edriss S Titi, and Shannon Wynne Abstract. We propose the viscous Camassa–Holm equations as a closure approximation for the Reynolds-averaged equations of the incompressible Navier-Stokes fluid. This approximation is tested on turbulent channel and pipe flows with steady mean. Analytical solutions for the mean velocity and the Reynolds shear stress for most of the flow region are consistent with experiments. We propose the viscous Camassa–Holm equations as a closure approximation for the Reynolds-averaged equations of the incompressible Navier-Stokes fluid. This approximation is tested on turbulent channel and pipe flows with steady mean. Analytical solutions for the mean velocity and the Reynolds shear stress for most of the flow region are consistent with experiments. A Connection Between the Camassa–Holm Equations and Turbulent Flows in Channels and Pipes Shiyi Chen, Ciprian Foias, Darryl D. Holm, Eric Olson, Edriss S. Titi, and Shannon Wynne Abstract. In this paper we discuss recent progress in using the Camassa– Holm equations to model turbulent flows. The Camassa–Holm equations, given their special geometric and physical properties, appear particularly well suited for studying turbulent flows. We identify the steady solution of the Camassa–Holm equation with the mean flow of the Reynolds equation and compare the results with empirical data for turbulent flows in channels and pipes. The data suggests that the constant α version of the Camassa–Holm equations, derived under the assumptions of isotropy and homogeneity, holds to order α distance from the boundaries. Near a boundary, these assumptions are no longer valid and the length scale α is seen to depend on the distance to the nearest wall. Thus, a turbulent flow is divided into two regions: the constant α region away from boundaries, and the near wall region. In the near wall region, Reynolds number scaling conditions imply that α decreases as Reynolds number increases. Away from boundaries, these scaling conditions imply α is independent of Reynolds number. Given the agreement with empirical and numerical data, our current work indicates that the Camassa–Holm equations provide a promising theoretical framework from which to understand some turbulent flows. In this paper we discuss recent progress in using the Camassa– Holm equations to model turbulent flows. The Camassa–Holm equations, given their special geometric and physical properties, appear particularly well suited for studying turbulent flows. We identify the steady solution of the Camassa–Holm equation with the mean flow of the Reynolds equation and compare the results with empirical data for turbulent flows in channels and pipes. The data suggests that the constant α version of the Camassa–Holm equations, derived under the assumptions of isotropy and homogeneity, holds to order α distance from the boundaries. Near a boundary, these assumptions are no longer valid and the length scale α is seen to depend on the distance to the nearest wall. Thus, a turbulent flow is divided into two regions: the constant α region away from boundaries, and the near wall region. In the near wall region, Reynolds number scaling conditions imply that α decreases as Reynolds number increases. Away from boundaries, these scaling conditions imply α is independent of Reynolds number. Given the agreement with empirical and numerical data, our current work indicates that the Camassa–Holm equations provide a promising theoretical framework from which to understand some turbulent flows.
更多查看译文
AI 理解论文
溯源树
样例
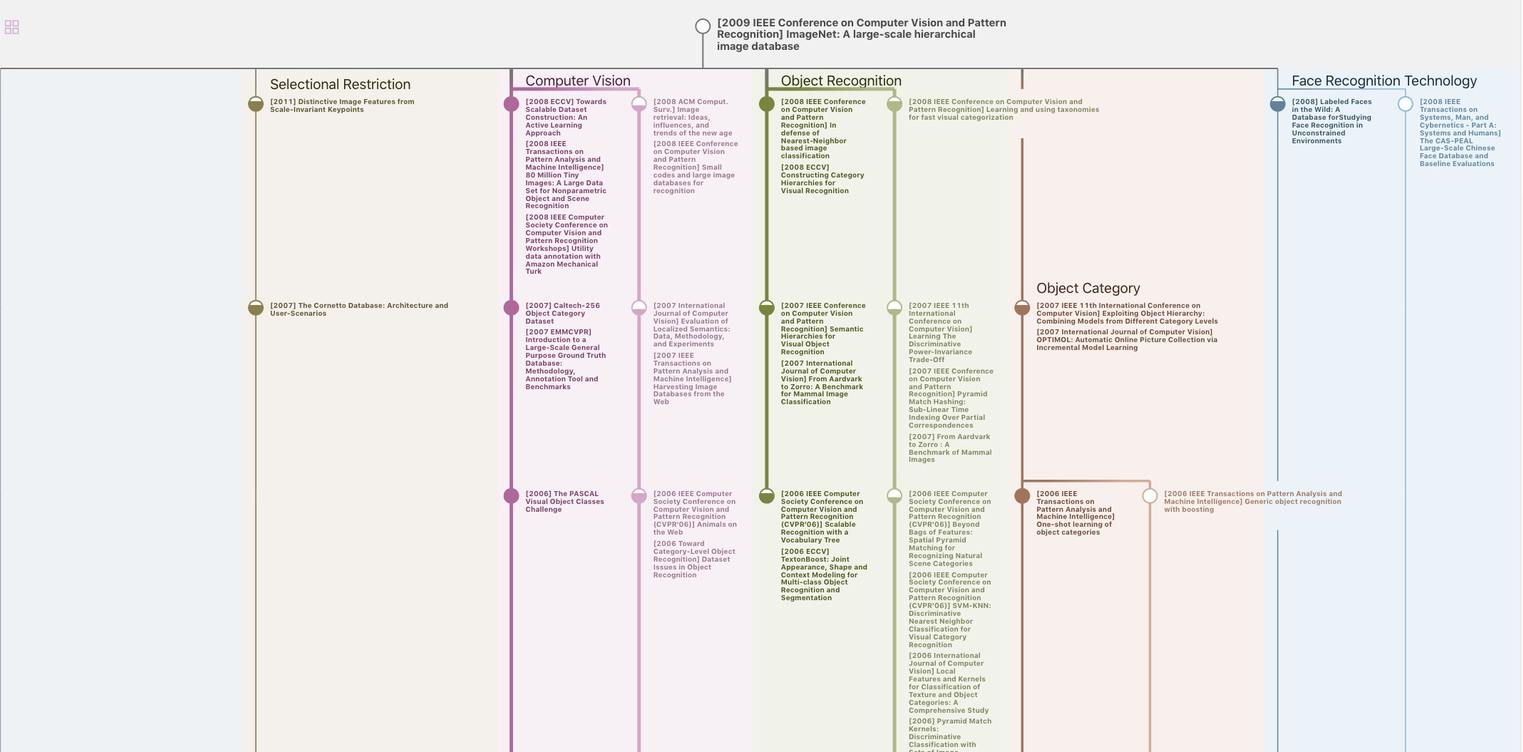
生成溯源树,研究论文发展脉络
Chat Paper
正在生成论文摘要