Effects of Forces Induced by Steady Streaming Flows on Rigid Oscillating Spheres
semanticscholar(2016)
摘要
A numerical method based on a perturbation approach is used to compute the microstreaming flow fields around a pair of spheres, along with the forces experienced on the spheres positioned at any arbitrary distance apart. The perturbation approach is first validated against a DNS and the literature. The aim is to determine the role of steady streaming causing particles to attract each other. Introduction Acoustics has recently been used to separate cream from milk [9]. When ultrasound is applied, a standing wave is formed in the fluid, which causes particles to collect at nodal or antinodal regions ([4], [6], [13]). In the case of milk, these particles are micron-sized, spherical, Milk Fat Globules (MFGs) that rise and separate as cream. The phenomenon of oscillating spheres travelling from nodes to antinodes in an acoustic field caused by the Direct Radiation Force (DRF) ([6], [13]) is well known to the responsible for this aggregation. It is also possible that the phenomenon of steady streaming around the oscillating spheres [12] also affects the aggregation. There is an extensive literature on DRF and their effects for spherical particles, but less attention is paid to miscrostreaming effects. The streaming flow around a sphere in a sound field was first analysed by C. A. Lane in 1955. Lane recognised that there are inner vortices in the boundary layer around a spherical particle that would drive outer vortices much bigger in size than the particle itself [8]. Longuet-Higgins [11] later computed steady streaming around spherical oscillating particles, but he did not take into account the forces involved. The focus of this work is to find the role of steady streaming in beneficially bringing particles together or detrimentally preventing the process, through a study of forces involved. In particular, we are interested in the threshold of frequency at which this process occurs. A numerical method based on perturbation approach is used to calculate forces exerted on two spheres. The method is first validated by a Direct Numerical Simulation (DNS) for streaming around a single sphere. Numerical Method We have used a perturbation method to calculate forces exerted by two spheres on each other solving the Weakly Non-Linear equations (WNL) in FreeFem++ [5]. In order to validate the perturbation method, we have first applied a full DNS for a single sphere of unit diameter D. An unbounded, incompressible fluid is assumed to be oscillating vertically with an amplitude A and frequency ω. For the case of two spheres, two stationary equisized spheres of unit diameter are fixed at a position with distance L apart and angle θ between their centers. A Cartesian (x,y,z) frame of reference is used together with a cylindrical (r,φ,z) frame. When θ = 0◦, the spheres are placed parallel to the axis of imposed oscillation, in an axial configuration. Similarly at θ = 90◦, the spheres are positioned perpendicular to the axis of imposed oscillation, in a lateral configuration. We defined the non-dimensional parameters, ε = A/D, described in the literature as inverse Strouhal number, and Reynolds number Re = AωD/ν, where D is the diameter, A is the amplitude and ω is the frequency of the oscillating flow, and ν is the kinematic viscosity. For the WNL approach applied for two spheres, a ratio of Re and ε is introduced as Ω = ωR2/ν, where R = D/2. In this case, we assumed that ε→ 0. Validation for the Case of a Single Sphere The DNS applied a spectral element method approach to solve the problem [10]. The numerical scheme integrated NavierStokes equations with respect to time using a mixed explicitimplicit time-splitting scheme based on backwards differentiation. A second order time variant of the method was employed for all calculations. The Gauss-Lobatto-Legendre quadrature was used for integration. Oscillating boundary conditions were applied on left and right sides for incoming and outgoing flow, with pressure gradient d p/dx= 0. At the top boundary, the flow was parallel (v = 0), with zero-stress (du/dy = 0), and the pressure gradient d p/dy = 0. In addition to a no-slip, the normal gradient of the pressure d p/dn = 0 at the surface of the sphere. We assumed an axi-symmetry over the axis of the oscillating
更多查看译文
AI 理解论文
溯源树
样例
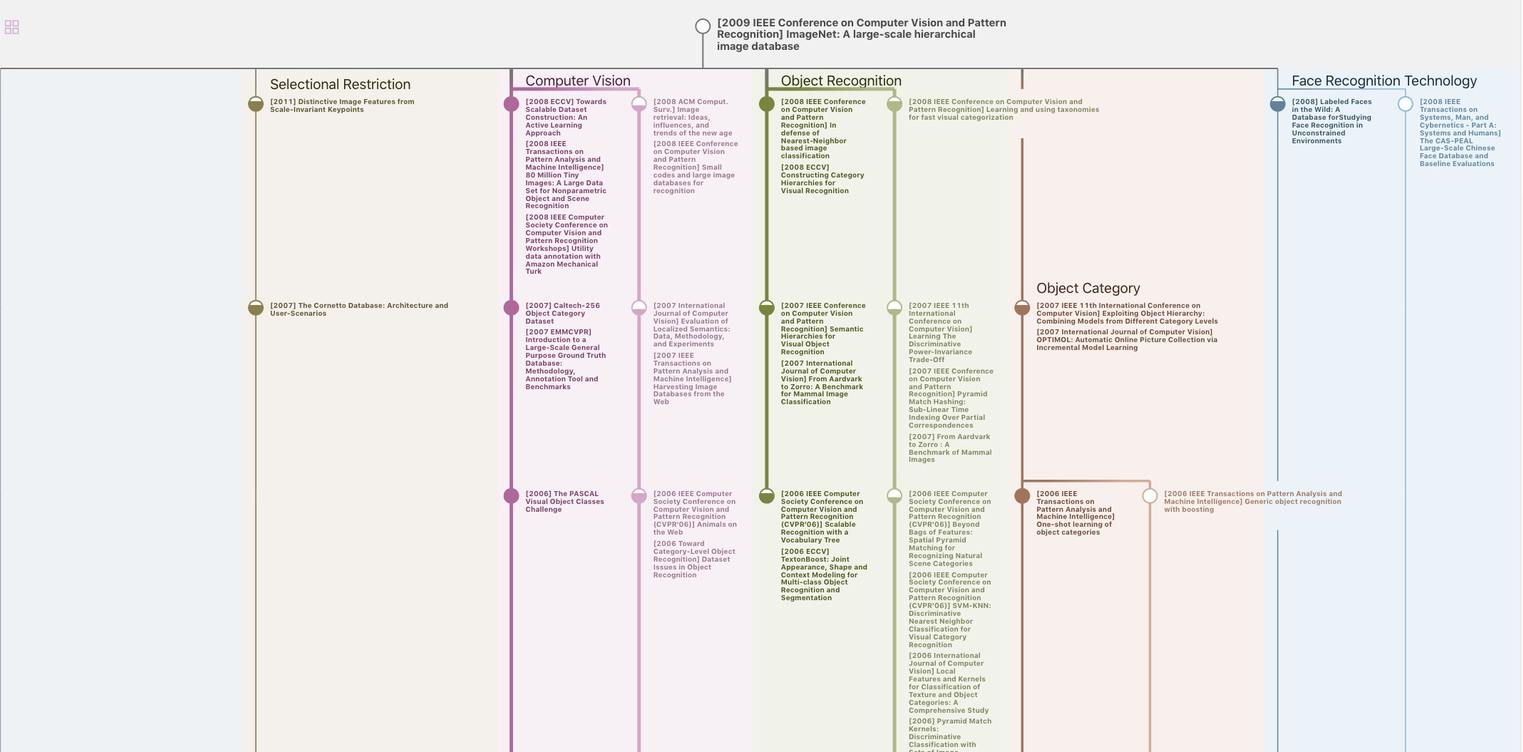
生成溯源树,研究论文发展脉络
Chat Paper
正在生成论文摘要