Characterization of the Microstructure and Thermoelectric Properties of Doped ZnO Ceramics
semanticscholar(2018)
摘要
Thermoelectric materials are known for their ability to convert a heat gradient into electrical power. They are currently gaining considerable attention for their potential applications in harvesting the large amounts of waste heat lost by industrial processes and automobiles. The performance of thermoelectric materials is characterized by the figure of merit, ZT, which is defined as ZT = σST/κ, where σ, S, κ and T are the electrical conductivity, Seebeck coefficient, thermal conductivity and temperature respectively. ZnO has been studied extensively for thermoelectric applications. ZnO has clear advantages for use as a thermoelectric including: low cost, use of abundant elements, nontoxicity and stability in air to temperatures in excess of 1000 C [1]. In this work, Mn-Al co-doped ZnO was studied in order to understand the effects of co-doping on the microstructure and thermoelectric properties of ZnO. Al is known to act as a donor in ZnO and can increase the electrical conductivity by up to three orders of magnitude [2]. Mn has a high solubility in ZnO (~8 at.% at 1400 C [3]) and can act as a point defect to scatter short wavelength phonons by substituting for Zn. This could potentially result in ZT improvement by a reduction of the thermal conductivity. Samples with the following stoichiometries: Zn1-x-yMnxAlyO (x = 0.0, 0.02, 0.05 and 0.1, y = 0.01) were prepared by sintering in air at 1400 C for 12 hr and also by spark plasma sintering (SPS) at 900 C for 30 min. Characterization was done by X-ray diffraction and SEM-EDS. Thermoelectric measurements were done by measuring the resistivity and Seebeck coefficient using the ZEM-2 (Ulvac Riko). The thermal conductivity was determined using the laser flash method. X-ray diffraction showed an increase in the lattice constants of ZnO for increasing Mn doping levels in the air-sintered sample [4]. This is attributed to the substitution of Mn on the Zn sublattice. Peaks due to a Zn1-xMnxAl2O4 spinel phase were also detected. Samples sintered by SPS at a lower temperature showed different secondary phase peaks. It was possible to index these peaks according to the hetaerolite Mn3-xZnxO4 phase, which is likely mostly due to unreacted Mn3O4. However, an increase of the lattice parameter of the ZnO phase was observed so it can be inferred that a finite amount of Mn substituted for Zn even at the low sintering temperature (900 C). SEM analysis of air-sintered samples showed a microstructure with precipitates dispersed throughout the grains and at grain boundaries. These precipitates were determined to be rich in Mn, Al and O and depleted of Zn relative to the ZnO matrix (Fig. 1), consistent with the results of XRD analysis. For high Mn doping levels (10 at.% Mn) segregation of Mn to grain boundaries with a corresponding depletion of Zn was detected. The electrical resistivity of the air-sintered samples (Fig. 2 (a)) increased in value and showed a gradual transition from metallic to semiconducting behavior for increasing Mn content. The thermal conductivity of air-sintered samples (Fig. 2 (b)) showed a trend
更多查看译文
AI 理解论文
溯源树
样例
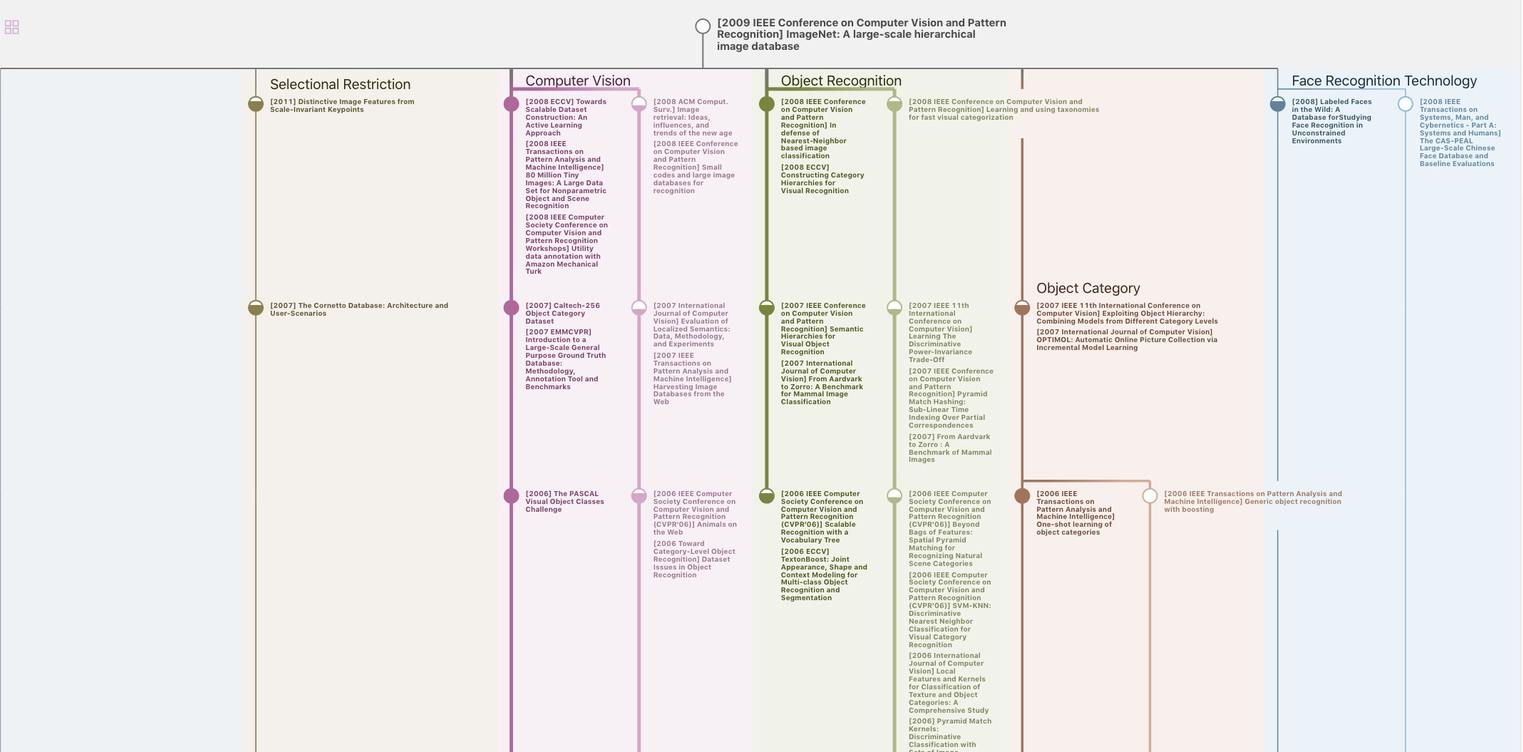
生成溯源树,研究论文发展脉络
Chat Paper
正在生成论文摘要