Dendrite‐Free Lithium Anode Via a Homogenous Li‐Ion Distribution Enabled by a Kimwipe Paper
Advanced sustainable systems(2017)
Abstract
Kimwipe (KW) paper is utilized, for the first time, to realize a dendrite-free Li-electrode surface. The polar functional groups of KWs facilitate a homogeneous Li-ion distribution and smooth Li redeposition. Therefore, placing a sheet of KWs on the top of a Li anode significantly improves the reversibility of Li anode. The metallic lithium (Li) anode has long been considered as the “Holy Grail” for establishing high-energy-density Li-metal based batteries (LMBs), such as Li-sulfur and Li-air batteries.1-6 The Li anode exhibits a high theoretical specific capacity of 3860 mA h g−1, the highest electrochemical reduction potential of −3.040 V (vs standard hydrogen electrode), and a low density of 0.59 g cm−3.3, 4 Nevertheless, the practical use of Li-metal anode suffers from an inherent challenge: the outgrowth of Li dendrites.1, 7 The Li dendrites are branched (or tree-like) Li deposits mainly arising from the highly nonuniform Li-ion redeposition on the electrode surface (Figure 1a).2-4, 8 The imperfect electrode surfaces (e.g., the Cu and Li electrodes shown in Figure S1, Supporting Information) with evident protrusions intensify the inhomogeneous Li-ion distribution due to the higher electric potential around these tips, which is named the “tip effect.”2, 4 The aggravated inhomogeneous Li-ion accumulation renders Li ions nonuniformly electrodeposit onto the electrode surface, producing an uneven Li deposit along with the germination of tree-like Li dendrites. The Li-dendrite sprouts and the nonuniform Li deposit cause the “self-amplification effect” that magnifies the inhomogeneity of Li-ion distribution and then promotes the outgrowth of Li deposits.2-4 Li dendrites protruding outward from the electrode surface could pierce through the polymeric separator, causing an internal short-circuit and even safety concerns.1, 3, 4 Additionally, the rough Li deposits with Li dendrites enlarge the surface area. The increased reactive surface accelerates the consumption of both Li and liquid electrolyte due to the formation of a solid-electrolyte interphase (SEI) layer.3, 9, 10 The accelerated reactions eventually deplete the electrolyte, thereby leading to battery failures. To address the issue of Li-anode instability, many strategies have been developed to inhibit the dendritic growth. Numerous electrolyte additives have been explored to inhibit the growth of Li dendrites by reinforcing the SEI layer on the Li surface. For example, hydrogen fluoride (HF),11 lithium fluoride (LiF),12 lithium nitrate (LiNO3),13 and 2-methylfuran (2Me-F)14 have been explored as additives. Although the electrolyte additives strengthen the SEI layer, the rapid consumption of additives upon cycling undermines their effectiveness in inhibiting the dendritic growth.15 On the other hand, the SEI layer with a lower modulus cannot sustain the huge volume change originating from the Li dendrite growth.2 In addition to these electrolyte additives, the ex situ artificial protections (e.g., tetraethoxysilane (TEOS),16 lithium nitride (Li3N) film,17 and composite coatings18) can also stabilize the Li anode. However, these remedies fail to solve the intrinsic problem leading to Li dendrites, that is, the inhomogeneous Li-ion distribution. In this regard, allowing Li ions to form a homogeneous distribution is the prerequisite for a dendrite-free electrode surface.3, 4 Recently, a smooth distribution of Li ions has been achieved by applying a membrane that has abundant polar functional groups on top of the Li anode, which inhibit the movement of Li ions toward protrusions induced by the tip effect.3, 4 A 3D oxidized polyacrylonitrile (PAN) nanofiber layer has been reported to immobilize Li ions onto polar functional groups (e.g., OH, CN, CO, and CN) and thereby prevent Li ions from gathering around protrusions, resulting in a smooth Li deposit.3 In another study, Zhang and co-workers used 3D glass fiber cloths with polar functional groups (e.g., SiO, OH, and OB) to facilitate an even distribution of Li ions and then achieve a dendrite-free surface.4 Inspired by this concept, we apply the Kimwipe (KW) paper on the surface of Li electrode to realize a dendrite-free surface and also to improve the reversibility of the Li anode. KWs, which are common laboratory supplies, are composed of a 3D fibrous framework of virgin wood fibers referred to as fresh cellulose fibers directly derived from plants. The cellulose fibers provide KWs with abundant polar functional groups to adhere to Li ions (Figure S2, Supporting Information) and, therefore, provide excellent wettability and impede the aggregation of Li ions around protrusions (Figure 1b).3, 4 As a result, Li ions homogeneously redistribute and then uniformly deposit on the electrode surface. These favorable features create a smooth Li deposit layer. Moreover, the electrically insulating cellulose fibers of KWs also pilot Li growth along fibers, allowing the epitaxial growth of Li onto the former Li layer due to the strong interaction between polar functional groups and Li ions.3, 4 Due to the homogeneous Li-ion distribution, the KWs effectively suppress the outgrowth of Li dendrites and ameliorate the cycling ability of Li anode. The dendrite-suppression behavior of KWs is mainly contributed from the abundant polar functional groups on their cellulose fibers. Fourier-transform infrared (FTIR) spectrum was employed to characterize the polar functional groups on the cellulose fiber of KWs. The building block of KWs is cellulose, linear polymers (poly-d-glucose) (Figure S3a, Supporting Information). The strong peaks at 3336 and 1635 cm−2, and 1056 cm−2 are attributed to, respectively, the stretching vibrations of polar OH groups and the COC stretching in poly-d-glucose chains.19 Unlike the Celgard separators composed of only nonpolar polymers, polypropylene (Figure S3b, Supporting Information), the polar KWs serve as an inhibitor to prevent Li ions from traveling toward the protrusions, where the enhanced electric field occurs. Moreover, the Brunauer–Emmett–Teller (BET) measurements demonstrate that KWs exhibit a surface area of 3.3 m2 g−1 (Figure S4, Supporting Information) which originates from the dense, layered porous structure consisting of entangled cellulose fibers (Figure S5, Supporting Information). Collectively, the KWs have both abundant polar functional groups and porous structures to improve the uptake of electrolyte, upgrade the electrolyte wettability, and offer a better electrode–electrolyte contact (Figure S6, Supporting Information).3 Therefore, a sheet of polar KWs inserted between the Li anode and the Celgard separator facilitates a homogeneous Li-ion distribution, thus forming a smooth Li deposit. The dendrite-inhibiting capability of KWs was assessed by Li-plating tests. For the purpose of making a clear and fair comparison, no electrolyte additives were used. The scanning electron microscopy (SEM) images (Figure 2) demonstrate the morphology of the deposited Li on the Cu foil. Li is plated on the Cu substrate at a current density of 3 mA cm−2 for 3 h. The control cell without the protection of KWs shows the nonuniform Li deposit with huge cracks that are possibly induced by the growth of Li dendrites (Figure 2a).7, 20 Figure 2b,c reveals that clear Li filaments with a diameter of ≈3 µm and a length of ≈20 µm randomly grown inside the cracks. This observation further confirms that the Li dendrites demolish the integrity of Li deposits. In sharp contrast to the unfavorable Li deposition with numerous dendrites, which results from the inhomogeneous Li-ion distribution, the KW-protected surface exhibits a smooth Li deposit without Li dendrites and shows the well-preserved integrity of the Li deposit (Figure 2d–f) at the same Li-plating condition. Moreover, Figure 2e shows that Li uniformly deposits within the cellulose-fiber framework of KWs and occupies the interspaces between the cellulose fibers of KWs. Continuously plating Li at an extremely high current density (100 mA cm−2) also clearly shows delayed internal short-circuits in the protected cell (Figure S7, Supporting Information), indicating the suppressed Li dendritic growth. The dendrite-free morphology and smooth surface in the KW-protected cell provide solid evidence of the homogeneous Li-ion distribution and stabilized Li electrodes. In addition to suppressing the growth of Li dendrites, KWs flatten the preexisting Li dendrites. To demonstrate this, Li filaments were intentionally pre-electrodeposited on the Cu electrode at a current density of 3 mA cm−2 for 3 h (Figure S8, Supporting Information). Subsequently, half of the electrode surface with predeposited Li dendrites remains unprotected as the control surface while the other half is protected by KWs as the experimental surface. The electrodeposition then underwent at the same current density for another 8 h. Figure S9a (Supporting Information) illustrates a clear contrast between the unprotected area and the protected area, evidencing the termination of the outgrowth of Li dendrites. The KW-protected area has relatively smooth surface (Figure S9b, Supporting Information), implying success in restoring the uneven predeposited Li.2 The homogeneous Li-ion redistribution due to the better Li-ion affinity to the polar KWs effectively transforms the rough surface into smooth surface. In contrast, the unprotected surface exhibits the pronounced roughness with Li filaments protruding outward (Figure S9c, Supporting Information). The inexorable dendrite formation is a result of the self-amplified nonuniform Li-ion distribution.2 Therefore, the distinctive surface morphologies in Figure S8 (Supporting Information) indicate that the KWs function as a matrix to interrupt the inhomogeneous accretion of Li ions on the electrode surface. The cross-sectional SEM images of the Li anode offer additional evidence for the suppressed dendrite growth. The Li anode was retrieved from the symmetric cells cycling at a current density of 2 mA cm−2 for 400 h. Figure S10a (Supporting Information) shows the porous structures and Li filaments on the Li anode of the control cell (Li||Li) without the protection with KW. The porous structure with Li dendrites increases the surface roughness and surface area, thereby accelerating the depletion of electrolytes due to the more vigorous reaction between the porous Li deposit and electrolytes during the repeated formation the SEI layer.1, 3, 4 Eventually, the complete depletion of electrolytes in the control cell leads to the cell failure. However, the KW-protected Li anode retrieved from the KW-protected cell (Li|KW||KW|Li) reveals the relatively compact Li deposit without any Li dendrites protruding outward (Figure S10b, Supporting Information). The compact deposition of Li restrains the rapid degradation of Li and electrolytes and, therefore, improves the cycling ability of the Li anode. The compact Li deposit reconfirms that the KWs with the abundant polar functional groups, including OH and COC groups, serve as the Li-ion redistribution layer to inhibit the inhomogeneous accretion of Li ions. Therefore, KWs facilitate the formation of the compact and dendrite-free Li deposit. The symmetric cell examination is a useful tool to investigate the reversibility of Li anode.12 Figure 3a illustrates the cell arrangements: the control cell (Li||Li) uses the bare Li foil as the electrode, while the KW-protected cell employs the Li foil covered with a sheet of KWs on its top as the electrode (Li|KW||KW|Li). The standard electrolyte contained 1.85 m lithium trifluoromethanesulfonate salt (LiCF3SO3) in 1,2-dimethoxyethane (DME)/1,3-dioxolane (DOL) cosolvent (volume ratio = 1:1). The symmetric cells with the standard electrolyte assembled with the 2032-type coin cell were cycled at various current densities of 2, 5, and 10 mA cm−2. As cycling proceeds, electrochemical deposition (Li plating) and Li dissolution (Li stripping) continuously undergo in the symmetric cells. The profiles of voltage versus cycling time are presented in Figure 3b–e. The negative potential results from the dissolution of Li, while the positive potential refers to the overpotential during the deposition of Li.7 Albeit the fresh protected cell exhibits a higher impedance (Figure S11, Supporting Information) due to the insulating KW papers, the protected cells completely outperformed the control cells in reversibility and stability. The control cell at a low current density of 2 mA cm−2 shows an increasing voltage polarization with unstable voltage fluctuations in the voltage versus cycling time profile during cycling (Figure 3b). The high volatility in the control cell is attributed to the nonuniform Li deposition with severe dendrite growth resulting from inhomogeneous Li-ion distribution.3 The polarization continuously escalates to the maximum value (≈700 mV) and then the cell fails in its normal operation after 400 h. The cell failure is mainly due to the complete consumption of electrolytes induced by the rapid reactions between porous Li deposits and electrolytes. In comparison, the KW-protected cell shows a stable cycling ability for 1000 h and a low polarization (≈200 mV) at the same current density of 2 mA cm−2 (Figure 3c). In addition, the KW-protected cells show promising reversibility at high current densities of 5 and 10 mA cm−2, respectively, for 1000 and 400 h (Figure 3d,e). At the high current density, the unfavorable dendrite growth is pronounced due to the increase in highly inhomogeneous Li-ion distribution.21 Thus, the superior cyclability of the KW-protected cell implies a homogeneous Li deposit and less consumption of both Li and electrolyte occurring in the cell. The extremely long-cycling span of 1800 h (75 d) at the current density of 5 mA cm−2 is also accomplished by means of the KW-protected cell (Figure S12, Supporting Information). Moreover, the KW-protected cells (Li|KW||KW|Li) outperform the control cell using the standard electrolyte containing the LiNO3 additive (LiNO3-Li||Li) (Figure S13, Supporting Information). The cycling stability of the control cells is significantly improved by using LiNO3 as an electrolyte additive at a current density of 2 mA cm−2 in the initial 1000 h. This is because LiNO3 facilitates the formation of reinforced SEI layer composed of ROCO2Li (R = alkyl groups), LiNxOy, CF3, and LiF, delaying the outgrowth of Li dendrites.3, 4, 13 Unfortunately, although LiNO3 has been reported to improve the cycling performance due to the formation of a high-quality SEI layer, our experimental results evidence that the LiNO3-Li||Li cell fails to survive in extending cycling life to >1000 h. The unfavorable drawback results from two main reasons. First, in view of the SEI layer, the LiNO3 only reinforces the relatively stable SEI layer with insufficient mechanical stability and it cannot stop the growth of Li dendrites. During long-term cell operation, the insufficient mechanical stability of the SEI layer and the unsolved inhomogeneous Li-ion distribution allow the outgrowth of dendritic Li, which devastates the formed SEI layer and the integrity of the Li deposit.15 Second, the protruding Li dendrites continuously react with the electrolyte and consume LiNO3, thereby reducing the effectiveness in suppressing Li dendrites.3 On the contrary, the KW-protected cell exhibits superior cycling performance with stable polarization after operating for 1200 h. The improved reversibility is attributed to the capability of KWs to distribute Li ions homogeneously on the surface rather than sacrificing themselves to produce a stable SEI layer. Therefore, the nonconsumable KWs not only effectively suppress the growth of Li dendrites, but also significantly enhance the reversibility of the Li anode for the extended cycling life. In summary, we utilized a facile method to improve the reversibility of Li anode by applying a sheet of KWs on the top of a Li anode. KWs possessing abundant polar functional groups serve as a media to facilitate a uniform Li-ion distribution on the electrode, avoiding the aggregation of Li ions around the protrusions. As a result, the homogeneous Li-ion distribution allows Li to deposit compactly and smoothly on the electrode surface, suppressing the formation Li dendrites. KWs also render any preexisting Li dendrites to be transformed into a dense and smooth Li layer. In symmetric cell examination, a stable cycling performance at various current densities of 2, 5, and 10 mA cm−2 is well-maintained for a long cycle life in a standard electrolyte (LiCF3SO3/DME-DOL) without any electrolyte additives. The stable cyclability demonstrates the effectiveness of KWs in inhibiting Li dendrites and enhancing the reversibility. This material is based upon work supported by the Department of Energy, Office of Energy Efficiency and Renewable Energy (EERE), under Award No. DE-EE0007218. The views and opinions of authors expressed herein do not necessarily state or reflect those of the U.S. Government or any agency thereof. As a service to our authors and readers, this journal provides supporting information supplied by the authors. Such materials are peer reviewed and may be re-organized for online delivery, but are not copy-edited or typeset. Technical support issues arising from supporting information (other than missing files) should be addressed to the authors. Please note: The publisher is not responsible for the content or functionality of any supporting information supplied by the authors. Any queries (other than missing content) should be directed to the corresponding author for the article.
MoreTranslated text
AI Read Science
Must-Reading Tree
Example
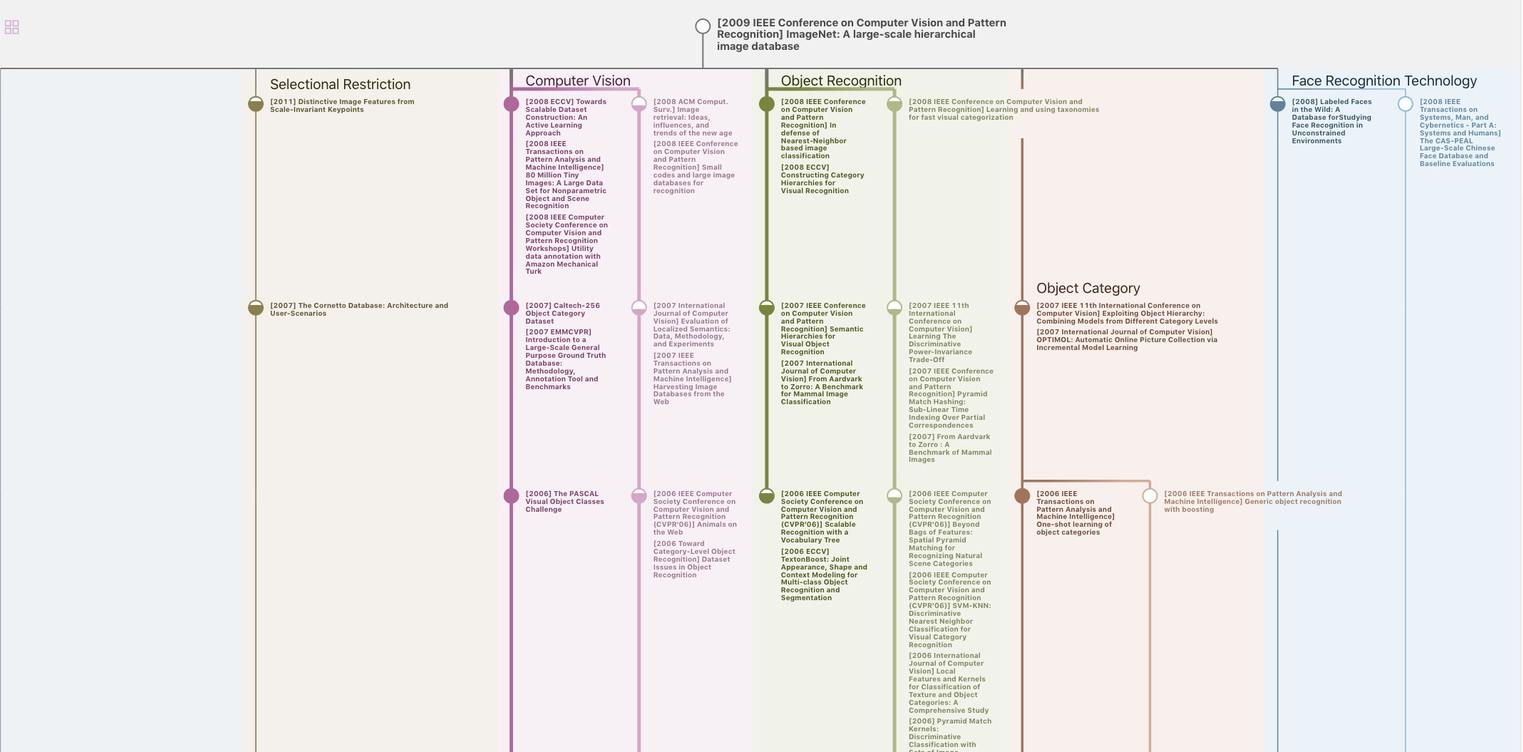
Generate MRT to find the research sequence of this paper
Chat Paper
Summary is being generated by the instructions you defined