O perando lab-Based X-Ray Computed Tomography of Zn-Air Batteries
user-5e9d449e4c775e765d44d7c9(2021)
摘要
In recent years, zinc-air (Zn-air) batteries have attracted increasing attention thanks to their high theoretical energy density, high relative abundance of materials, safety and relative low cost, compared with lithium counterparts1. Whilst they have been widely used in hearing aid technologies2, Zn-based technologies are considered promising for grid-based storage applications and back-up power2. However, challenges still remain for the wide-range adoption of the technology, including challenges facing finding good bi-functional catalysts, avoiding dendrite growth on the Zn anode and increasing cyclability and lifetime. Whilst there is a significant research focus on improved cathode materials, less work has focused on the complex charge/discharge reactions of the zinc anode 3. Thus, this work has a particular focus on characterisation and electrode engineering of the anode. X-ray computed tomography (CT), which is a non-destructive 3D imaging technique capable of imaging inside samples, has been widely used in recent years for imaging energy materials4. A significant advantage of the technique is the ability to carry out 4D studies (i.e. three spatial dimensions plus time), which allows for morphological evolution to be elucidated during device operation. Thus, structural features (like dendrites, or the conversion of zinc to zinc oxide) can be visualised and correlated to electrochemical performance. For Zn-air batteries, X-ray CT has been used in a number of studies, although a greater number have used synchrotron X-ray sources than lab-based X-ray instruments5–7. This work will discuss the development of bespoke Zn-air cells suitable for correlative, operando characterisation using lab-based techniques, where 3- and 4D imaging methods, like X-ray CT, are combined with electrochemical studies to deepen the understanding of the reactions of zinc and zinc-oxide during cell discharge and charge, respectively. Electrochemical cell design for operando imaging is a particular challenge, since there is a trade-off between obtaining good electrochemical performance and having a cell whose size is suitable for resolving the desired features of interest in the sample. As shown in Figure 1a), the cell design is relatively simple and transport of air to the cathode occurs via holes milled into the casing. Furthermore, the cell design is highly versatile and can be easily adapted to suit the desired imaging resolution. The ability to carry out multiscale imaging is a particular advantage, given that features of Zn-air cells range from the mm thick electrodes to μm/nm-size particles. Whilst 2D techniques like SEM can only determine surface information about a sample, X-ray CT can examine the interfaces between materials, which is particularly important for understanding how zinc particles evolve during cell operation. The cell sits between a source and detector (Figure 1b), on a rotating stage and the resulting 3D volume can be analysed by segmentation of individual features. Shown in Figure 1c is an example volume of a zinc anode. The particles of zinc can clearly be observed on the top of the carbon paper substrate (Figure 1c). By separating the individual particles and assigning each individual zinc particle a unique number (represented in Figure 1d by the various colours), a range of metrics can be determined, such as number of particles or particle volume distribution (Figure 1e). Insights gained through operando imaging experiments, including a deepened knowledge of the migration and transformation of individual zinc particles, can then be translated into improved electrode design. 1 P. Gu, M. Zheng, Q. Zhao, X. Xiao, H. Xue and H. Pang, J. Mater. Chem. A, 2017, 5, 7651–7666. 2 V. Caramia and B. Bozzini, Mater. Renew. Sustain. Energy, 2014, 3, 28. 3 J. Zhang, Q. Zhou, Y. Tang, L. Zhang and Y. Li, Chem. Sci., 2019, 10, 8924–8929. 4 T. M. M. Heenan, C. Tan, J. Hack, D. J. L. Brett and P. R. Shearing, Mater. Today, 2019, 31, 69–85. 5 D. Stock, C. Pompe and D. Schröder, Chemie-Ingenieur-Technik, 2019, 91, 555–559. 6 M. K. Christensen, J. K. Mathiesen, S. B. Simonsen and P. Norby, J. Mater. Chem. A, 2019, 7, 6459–6466. 7 V. Yufit, F. Tariq, D. S. Eastwood, M. Biton, B. Wu, P. D. Lee and N. P. Brandon, Joule, 2019, 3, 485–502. Figure 1
更多查看译文
AI 理解论文
溯源树
样例
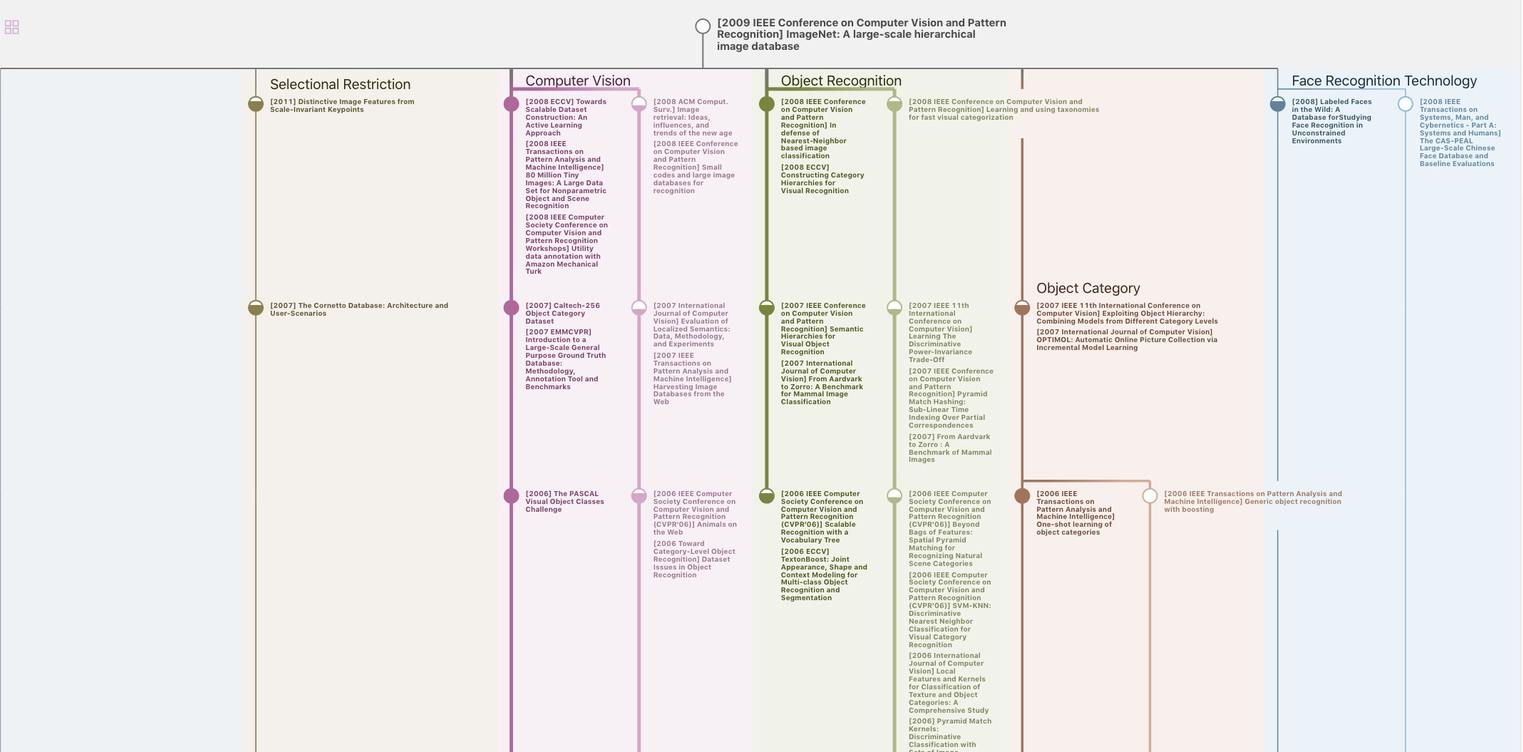
生成溯源树,研究论文发展脉络
Chat Paper
正在生成论文摘要