TERTing the Hyperoxic Lung.
American journal of physiology Heart and circulatory physiology(2021)
Abstract
Editorial FocusTERTing the hyperoxic lungVigneshwaran Vellingiri and Dolly MehtaVigneshwaran VellingiriDepartment of Pharmacology and Regenerative Medicine, University of Illinois, College of Medicine, Chicago, Illinois and Dolly MehtaDepartment of Pharmacology and Regenerative Medicine, University of Illinois, College of Medicine, Chicago, IllinoisPublished Online:23 Nov 2021https://doi.org/10.1152/ajpheart.00580.2021This is the final version - click for previous versionMoreSectionsPDF (760 KB)Download PDF ToolsExport citationAdd to favoritesGet permissionsTrack citations ShareShare onFacebookTwitterLinkedInWeChat Acute respiratory distress syndrome (ARDS), characterized by build of edema fluid in the lungs, leads to impaired alveolar oxygenation causing 40% lethality in the intensive care unit (ICU), including in patients experiencing severe COVID-19 (1). Mechanical ventilation with high-oxygen content remains a life-saving intervention in hospitalized patients with ARDS and COVID-ARDS due to lack of effective therapeutic options (2). Paradoxically, prolonged high oxygen breathing leads to severe hyperoxic acute lung injury that further worsens respiratory compliance and patients’ survival from ARDS. Therefore, identifying critical signaling nodes triggering oxygen toxicity during hyperoxia is needed to prevent lung damage. Hyperactivation of several signaling pathways and cross talk between during hyperoxia are thought to govern the lung damage (3). Lung vascular endothelial cells (ECs) generate reactive oxygen species (ROS) under hyperoxia that apparently contribute to barrier dysfunction. It is now established that endothelial mitochondria dynamically regulates signaling cellular responses to oxygen. Consistently, studies have shown an important role of mitochondrial dysfunction in the etiology of hyperoxia-induced lung injury in both large and small animal models of injury (3). Autophagy and mitophagy are important cellular processes that by breaking down cellular contents preserve energy and safeguard cells against accumulation of damaged and aggregated biomolecules (4). In this context, evidence indicates that during hyperoxia both autophagy and mitophagy may be activated in ECs to remove the dysfunctional mitochondria (3). Telomerase reverse transcriptase (TERT), a catalytic subunit of the enzyme telomerase, which, together with the telomerase RNA component (TERC), comprises telomerase complex to elongate chromosomal ends (5). While TERT is highly expressed and active in dividing cells, it is also expressed in differentiated cells, such as ECs. Thus, various contemporary studies have demonstrated the nontelomeric functions of this enzyme. For example, the loss of TERT resulted in microvascular damage leading to coronary arterial disease (6). Specifically, regressed TERT activity in ECs shortened telomere length leading to altered gene expression and decreased life span. Introduction of TERT restored endothelial functions, raising a possibility that TERT attrition may be a primary abnormality that renders the organism more susceptible to the vascular pathologies (6, 7). In this context, Ale-Agha et al. (8) generated mice models expressing TERT only in mitochondria (mitoTERT) or nucleus (nucTERT) to delineate the role of TERT after ischemia-reperfusion injury. These authors showed that mitoTERT preserved cardiac functions in ischemic mice in part through enhancing migratory capacity of ECs and activation of endothelial nitric oxide synthase. Aberrant ROS production may induce the export of nuclear TERT into the cytosol. Concomitantly, binding of TERT to mitochondrial DNA protected them from oxidative damage. TERT was also shown to induce conversion of LC3B I to LC3B II in fibroblasts, indicative of increased autophagosome formation. Thus, mitochondrial TERT and not nuclear TERT seems to protect mitochondria function during injury. Whether TERT integrates with mitophagy and autophagy to preserve microvascular function during hyperoxia remained unclear.In a recent issue of the American Journal of Physiology-Heart and Circulatory Physiology, Beyer et al. (9) by a series of studies unraveled a novel link between TERT and autophagy in response to hyperoxia-induced vascular injury. The authors showed that hyperoxia impaired autophagy signaling and decreased TERT expression in association with mitochondrial damage and inflammation in rat lungs. The authors also showed that hyperoxia-induced mitochondrial damage was more pronounced in rat lungs lacking catalytic subunit of TERT than in wild-type (WT) mice. Impairment of TERT activity reduced EC proliferation rather than death. However, these responses seem to occur independent of alterations in mitophagy, since the expression of Pink1 and Parkin were similarly altered in lungs from hyperoxic WT or TERT knockout rats. Further studies revealed that TERT and autophagy pathway operate in parallel to protect mitochondria from hyperoxic injury. Notably, EC activation of TERT and autophagy strengthened EC barrier function despite hyperoxia, suggesting targeting TERT and autophagy can be touted to promote vascular repair during hyperoxia.Although these new findings are highly informative, the study has raised some important questions. The mechanism by which TERT and autophagy enhanced EC barrier function is unclear. Is it simply the reversal of mitochondrial dysfunction or engagement of barrier protective pathways through TERT and autophagy? In addition, TERT and autophagy regulation of barrier function in vitro versus in lungs suggest that more complex mechanisms may be at work. For example, noncanonical role of mitochondria in regulating VE-cadherin assembly have been described recently (5). Autophagosomes sequester dysfunctional organelle, which then fuses with acidic lysosomes for their degradation (4). Beyer et al. (9) also showed that the loss of TERT function impaired clearance of autophagosome during hyperoxia. Whether it involved regulation of lysosomal hydrolase by TERT needs to parsed out. In addition, caspases directly interact with core autophagy machinery. Caspase-9 is involved in the intrinsic mitochondrial death pathway. Caspase-9 cleaves and activates downstream effector caspases, such as caspase-3, which can eventually lead to endonuclease degradation of DNA (10). Therefore, it would be rewarding to study whether impaired autophagic clearance in TERT was associated with the activation of caspase signaling. The accumulation of dysfunctional mitochondria is a shared hallmark of aging (7). It is well known that lung injury compromises the survival of older than younger patients (1). The finding of Beyer et al. raises an interesting question whether augmenting TERT expression or autophagy in these patients could increase survival from the deadly diseases.Hyperoxia-mediated mitochondrial dysfunction and ensuing oxidative stress have a potential to form a vicious cycle causing endothelial dysfunction that is critical to the pathogenesis of ARDS. Although more detailed studies are warranted, the work by Beyer et al. does provide insights into the role that TERT plays in linking mitochondrial dynamics with the lung vascular homeostasis. Nevertheless, the authors provide rationale for employing TERT and autophagic-specific pharmacological compounds in animal models of hyperoxia-induced lung injury to delineate the mechanisms regulating mitochondrial dysfunctions and its impact on endothelial barrier functions (Fig. 1). Therefore, the findings obtained holds promise to improve therapies targeting ARDS and many forms of age-related diseases.Figure 1.Model of hyperoxia-induced endothelial cell signaling. Hyperoxia reduces mitochondrial dynamics and autophagic capacity that leads to excess reactive oxygen species generation (ROS). Hyperoxia also reduces telomerase reverse transcriptase (TERT) activity. Together these events lead to inflammation and loss of endothelial barrier integrity. Treatment with telomerase increasing compound, AGS-499, or autophagy activator, trehalose, reduces hyperoxia-induced mitochondrial dysfunction restoring endothelial barrier function. MPO, myeloperoxidase; TSA, trichostatin A. Created with Biorender.com and used with permission.Download figureDownload PowerPointGRANTSThis work was supported by National Heart, Lung, and Blood Institute Grants HL84153 and HL137179.DISCLOSURESNo conflicts of interest, financial or otherwise, are declared by the authors.AUTHOR CONTRIBUTIONSV.V. prepared figures; V.V. and D.M. drafted manuscript; D.M. edited and revised manuscript; D.M. approved final version of manuscript.ACKNOWLEDGMENTSWe acknowledge Drs. Reddy and Wary (University of Illinois at Chicago) for comments.REFERENCES1. Matthay MA, Zemans RL, Zimmerman GA, Arabi YM, Beitler JR, Mercat A, Herridge M, Randolph AG, Calfee CS. Acute respiratory distress syndrome. Nat Rev Dis Primers 5: 18, 2019. doi:10.1038/s41572-019-0069-0.Crossref | PubMed | ISI | Google Scholar2. Pinhu L, Whitehead T, Evans T, Griffiths M. Ventilator-associated lung injury. Lancet 361: 332–340, 2003. doi:10.1016/S0140-6736(03)12329-X.Crossref | PubMed | ISI | Google Scholar3. Kluge MA, Fetterman JL, Vita JA. Mitochondria and endothelial function. Circ Res 112: 1171–1188, 2013. doi:10.1161/CIRCRESAHA.111.300233.Crossref | PubMed | ISI | Google Scholar4. Levine B, Kroemer G. Biological functions of autophagy genes: a disease perspective. Cell 176: 11–42, 2019. doi:10.1016/j.cell.2018.09.048.Crossref | PubMed | ISI | Google Scholar5. Mameli E, Martello A, Caporali A. Autophagy at the interface of endothelial cell homeostasis and vascular disease. FEBS J. 2021 May 2. doi:10.1111/febs.15873. Epub ahead of print.Crossref | PubMed | ISI | Google Scholar6. Kurz DJ, Decary S, Hong Y, Trivier E, Akhmedov A, Erusalimsky JD. Chronic oxidative stress compromises telomere integrity and accelerates the onset of senescence in human endothelial cells. J Cell Sci 117: 2417–2426, 2004. doi:10.1242/jcs.01097.Crossref | PubMed | ISI | Google Scholar7. Green DR, Galluzzi L, Kroemer G. Mitochondria and the autophagy-inflammation-cell death axis in organismal aging. Science 333: 1109–1112, 2011. doi:10.1126/science.1201940.Crossref | PubMed | ISI | Google Scholar8. Ale-Agha N, Jakobs P, Goy C, Zurek M, Rosen J, Dyballa-Rukes N, Metzger S, Greulich J, von Ameln F, Eckermann O, Unfried K, Brack F, Grandoch M, Thielmann M, Kamler M, Gedik N, Kleinbongard P, Heinen A, Heusch G, Gödecke A, Altschmied J, Haendeler J. Mitochondrial telomerase reverse transcriptase protects from myocardial ischemia/reperfusion injury by improving complex I composition and function. Circulation. 2021 Oct 21. doi:10.1161/CIRCULATIONAHA.120.051923. Epub ahead of print.Crossref | PubMed | ISI | Google Scholar9. Beyer AM, Norwood Toro LE, Hughes WE, Young M, Clough AV, Gao F, Medhora M, Audi SH, Jacobs ER. Autophagy, TERT, and mitochondrial dysfunction in hyperoxia. Am J Physiol Heart Circ Physiol 321: H985–H1003, 2021. doi:10.1152/ajpheart.00166.2021.Link | ISI | Google Scholar10. Cui D, Sun D, Wang X, Yi L, Kulikowicz E, Reyes M, Zhu J, Yang ZJ, Jiang W, Koehler RC. Impaired autophagosome clearance contributes to neuronal death in a piglet model of neonatal hypoxic-ischemic encephalopathy. Cell Death Dis 8: e2919, 2017. doi:10.1038/cddis.2017.318.Crossref | PubMed | ISI | Google ScholarAUTHOR NOTESCorrespondence: D. Mehta ([email protected]edu). Download PDF Previous Back to Top Next FiguresReferencesRelatedInformation Collections Related ArticlesAutophagy, TERT, and mitochondrial dysfunction in hyperoxia 27 Oct 2021American Journal of Physiology-Heart and Circulatory PhysiologyCited ByState of the field: cellular and exosomal therapeutic approaches in vascular regenerationEvan Paul Tracy, Virginia Stielberg, Gabrielle Rowe, Daniel Benson, Sara S. Nunes, James B. Hoying, Walter Lee Murfee, and Amanda Jo LeBlanc24 March 2022 | American Journal of Physiology-Heart and Circulatory Physiology, Vol. 322, No. 4 More from this issue > Volume 321Issue 6December 2021Pages H1103-H1105 Crossmark Copyright & PermissionsCopyright © 2021 the American Physiological Society.https://doi.org/10.1152/ajpheart.00580.2021PubMed34738834History Received 27 October 2021 Accepted 4 November 2021 Published online 23 November 2021 Published in print 1 December 2021 KeywordsARDSautophagyhyperoxialungTERT Metrics
MoreTranslated text
Key words
High-flow Oxygen Therapy
AI Read Science
Must-Reading Tree
Example
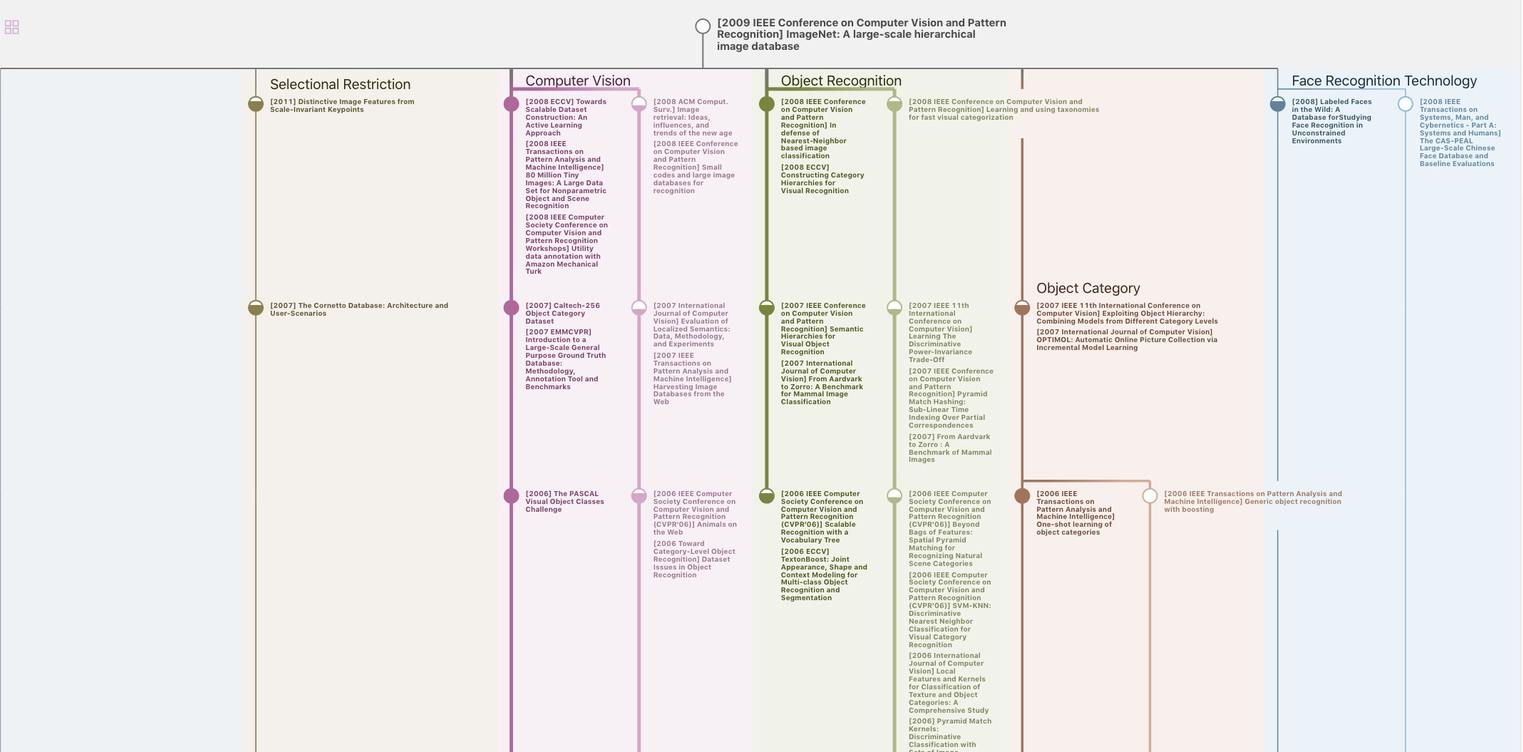
Generate MRT to find the research sequence of this paper
Chat Paper
Summary is being generated by the instructions you defined