How Compound-Wave Control Alleviates Hyper-Redundant Control Complexity
semanticscholar(2016)
摘要
Snakes use a variety of modes of locomotion to negotiate with challenging terrain, rendering them the superior capability to traverse unstructered environment inaccessible to conventional locomotor. Motivated by this advantage related with limbless locomotion, many research groups have been developing snake robots that have this superior mobility, which presents in their biological counterpart. However, to emulate this naturally existent redundant locomotor, the cost of such versatility is possessing many degrees of freedom and seamlessly coordinating them to produce desired workspace motion. This demanding requirement on resolution of degrees of freedom (joint angles) complicates the robot’s engineeringl design, makes motion planning unintuitive, and increases control complexity. To address these difficulties, the robotics community has derived parameter-based controllers with reduced dimensionality that naturally coordinate many degrees of freedom such as the serpenoid curves. In robotics, the planar version of the serpenoid curve was already extended into three dimensions, creating what we refer to as the compound serpenoid curve, which makes it possible to model biologically inspired snakelike motions, such as sidewinding, which have in-plane as well as out-of-plane motion. Moreover, physical controllers utilizing this parameterization for snake robots have been able to induce behaviors similar to that of the robots’ biological counterparts. This is an advance over the traditional serpenoid curve, which can only be used to model predominantly in-plane motions like slithering. While the serpenoid curve and compound serpenoid curve models have existed in the robotics community for some time, a very similar concept, known as control template, has co-existed within the biological locomotion community and is used in the study of animal movements. In biology, a control template is defined in terms of a model of a behavior that “contains the smallest number of variables and parameters that exhibits a behavior of interest”. Therefore, as in robotics, control templates in biology make it possible to use low-dimensional models to represent and subsequently study various aspects of biological motion control. So far, however, the template model in biology has mostly been restricted to planar locomotion and thus is not sufficient to capture a large subset of biological locomotor modes. Research of our group mainly focuses on snake robot locomotion. One of our research interest is dimensionality reduction for hyper redundant mechanism to reduce control and planning complexity. Research on controlling snake robots sheds light on natural control law for biological snakes and inspires biological investigation on animal locomotion behavior and control strategies. For example, the compound serpenoid curve for modular snake robots is extended to three-dimensional biological control 1X. Xiao, J. Dai, W. Zhen, C. Gong and H. Choset are with the Robotics Institute at Carnegie Mellon University, Pittsburgh, PA 15213, USA. {xuesuxiao@, jind@, weikunz@andrew., chaohuig@, choset@}cmu.edu 2H. C. Astley and D. I. Goldman are with the School of Physics, Georgia Institute of Technology, Atlanta, GA 30332, USA. {henry.astley, daniel.goldman}@physics.gatech.edu Fig. 1. (A) Differential and (B) reversal turning in a sidewinder rattlesnake. (C) Differential and (D) reversal turning implemented on a snake robot. (E) Demonstration of frequency turning. templates, which we refer to as the compound-wave control template. The purpose of this extension is twofold. First, we wish to provide a means for biologists to better model and examine the three-dimensional behaviors exhibited by limbless locomotion systems, e.g., sidewinding snakes. Second, biological intuition from control templates provides us with heuristics for low-dimensional motion design for snake robots, enhancing preexisting capabilities as well as designing entirely new behaviors. We validate the results by applying the compound wave control templates of biological systems on a snake robot, using the parameters from the control template in the compound serpenoid model to generate the joint trajectories necessary to replicate the biological turning (differential, reversal, and frequency turning) behavior (Fig. 1). Based on the compound serpenoid curve for snake robots, a technique called Locomotive Reduction is proposed, which reduces the control complexity of a 16-DoF snake robots to that of simply navigating a differential-drive car. This robotics technique also serves as a hypothesis of the control strategy for the steering and navigating behavior of biological snakes. In addition to these current results, our group is still using robotics techniques to investigate biological snake behaviors, e.g., responses of snakes to varying heterogeneous environment. For example, how biological sidewinders regulate their body shape and contact with substrate when facing obstacles. This is nontrivial since sidewinders cut a broad swath through the environment when moving and is confronted with a higher probability of collision. The decision making on different collision avoidance strategies is of interest and difficult to investigate from biological side, which, however, is relatively more straightforward in robotics, i.e. through optimization based on certain cost related with executing corresponding dodging strategies.
更多查看译文
AI 理解论文
溯源树
样例
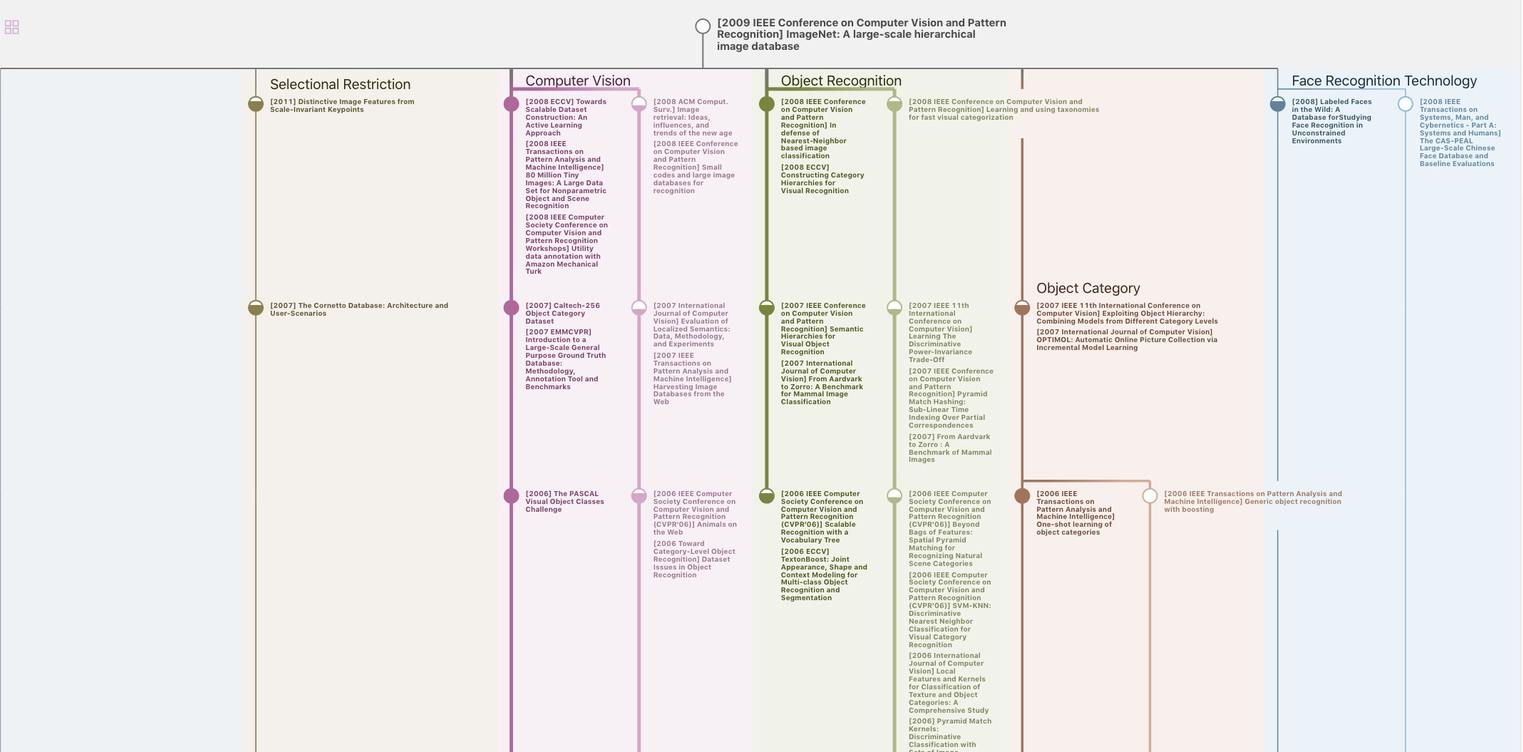
生成溯源树,研究论文发展脉络
Chat Paper
正在生成论文摘要