Nanoscale electronic transparency of wafer-scale hexagonal boron nitride
semanticscholar(2021)
摘要
Monolayer hBN has attracted interest as a potentially weakly interacting 2D insulating layer in heterostructures. Recently, wafer-scale hBN growth on Cu(111) has been demonstrated for semiconductor chip fabrication processes and transistor action. For all these applications, the perturbation on the underlying electronically active layers is critical. For example, while hBN on Cu(111) has been shown to preserve the Cu(111) surface state 2D electron gas, it was previously unknown how this varies over the sample and how it is affected by local electronic corrugation. Here, we demonstrate that the Cu(111) surface state under wafer-scale hBN is robustly homogeneous in energy and spectral weight over nanometer length scales and over atomic terraces. We contrast this with a benchmark spectral feature associated with interaction between BN atoms and the Cu surface, which varies with the Moiré pattern of the hBN/Cu(111) sample and is dependent on atomic registry. This work demonstrates that fragile 2D electron systems and interface states are largely unperturbed by local variations created by the hBN due to atomic-scale interactions with the substrate, thus providing a remarkably transparent window on low-energy electronic structure below the hBN monolayer. Background and Motivation Monolayers of hexagonal boron nitride (hBN) have emerged as a valuable building block within the burgeoning materials class of van der Waals (vdW) heterostructures. Applications for hBN vary and include active-layer spacing and isolation1–3, softening self-gating effects from trapped charge1,4–7, finessing twist-angle engineering8–10, and passivation of fragile or ambient-sensitive surfaces11–13. These materials and devices can include exfoliated or as-grown hBN. While many such devices explore new ground states involving the emergence of electronic order, magnetism, or superconductivity, there are nearer term efforts in the semiconductor industry to exploit 2D insulators—for which hBN has arisen as the prototype—within conventional silicon-based chip technology14,15. In these nanotechnology efforts, CVD growth is preferred for large-area coverage and compatibility with both metallic and insulating substrates. Regardless of the time horizon of research and development, it is critical to understand how the simplest insulator affects the electronic structure of the element it covers in the fabrication process. Toward this end, we examine one of the cleanest large-area insulator-metal systems: the interface between a monolayer of hBN and a two-dimensional electron system (2DES). The hBN monolayers are grown on Cu(111) using a new wafer-scale growth process developed in the semiconductor industry16. Cu(111) on sapphire provides an isolated and natural 2DES which exists as a surface state trapped between a Cu band gap along the [111] direction on the substrate side, and the insulating (vacuum or hBN) interface on the other side. Although hBN can be grown on other metals such as Ir17,18, Rh19, Ru20 , Cr21, Fe22, Ni23–25, Pd26,27 , Pt28,29, we have chosen the Cu(111) system because of its demonstrated compatibility with wafer-scale technology and because its surface state is exceeding well characterized. The 2D electrons within this surface state have been used for a variety of fundamental experiments involving coherent quantum nanostructures and atom manipulation accessing quantum phase30, quantum spin31, and designer quantum materials32. In principle the as-grown hBN/Cu(111) interface should be a benchmark for electronic homogeneity. Furthermore, hBN has been proposed as a Cu interconnect diffusion barrier to protect neighboring active regions on chip33, and hence has promise as a passivation or protection layer on complex quantum materials. Since these materials typically must be studied with the hBN in place, its perturbation on the underlying quantum material must be considered. In this study we have used scanning tunneling microscopy and spectroscopy to gather large spectral maps of the Cu(111) 2DES and neighboring spectral features so that statistical variations in the surface state and neighboring Moiré spectral features can be quantified and spatially indexed to the hBN-Cu(111) Moiré unit supercell. Results and Discussion The Moire pattern arising from the lattice mismatch and rotation angle between Cu(111) and hBN is seen in Fig. 1a,c,d, acquired in constant-current mode at Vs = 4V and Is = 167 pA. The Moire wavelength shown here is ƛ = 3.0 nm, corresponding to a rotation angle φ ≈ 4.6° assuming a lattice mismatch of 2.2%16. This pattern persists over multiple Cu step edges, with the orientation of the Moire pattern and A, B, and C sites preserved as well, consistent with single crystalline character of the hBN monolayer. In Fig. 1b (Vs = -2.5 V and Is = 33 pA) we see atomic resolution of the clean hBN monolayer. We identify three sites of interest as labelled in Fig. 1a inset: the bright spots of the Moire pattern (site A), and sites between the bright spots, one less dark (site B) and one more dark (site C). Each of these sites forms a triangular lattice of the same period, consistent with a pattern arising from different atomic registries. As detailed below, the A sites are associated with an N atom on top of a copper atom and B in an fcc position (NtopBfcc) as suggested in previous work on morphology of hBN/Cu(111) systems7,34, appearing as bright spots in the Moire pattern at Vs = 4V due to a the CBM being closer to the Fermi level for this registry, leading to a larger local density of states. The dI/dV features of the hBN/Cu(111) system are dominated by the hBN bandgap as shown in Fig. 2a). The bandgap is ~6 eV but can vary locally by hundreds of meV, in agreement with past STM, ARPES, and spectroscopic measurements7,35. At lower junction resistance we can probe features within the gap. For dI/dV spectra taken at a current setpoint Is = 167 pA and a stabilization voltage Vs = -700 mV (well within the hBN band gap) we see two consistent features which we highlight in Figs. 2b-c). Figure 2b shows a peak in the differential conductance at near -530 mV. This feature appears on multiple regions of the sample, but is not a feature that appears in dI/dV on a bare Cu(111) surface36, implying it appears only due to the presence of the hBN layer on Cu(111). To investigate this energy we fit this peak to a Gaussian and find the maximum, and label this energy EBN. The other feature highlighted in Fig. 2c shows the characteristic broadened stepedge line shape of the Shockley-type Cu(111) surface state, as observed in other STM work on hBN/Cu(111) systems7,34. The intact surface state suggests the interaction between the Cu(111) surface and hBN monolayer is sufficiently weak so that the surface state remains intact, and we are tunneling through the hBN layer directly into the Cu(111) surface state. We fit the midpoint of the rising side of the broadened step function, representing the onset of the surface state, and label this energy E2DES. We investigate the behavior of the EBN and E2DES over multiple terraces of hBN on Cu(111) as shown in Fig. 2d-f. These terraces are separated by over a micron, but each shows the Moire pattern characteristic of monolayer hBN on Cu(111). On each terrace we acquired ~1000 dI/dV spectra over a 5nm x 5nm area, and fit the spectra as described above to find EBN and E2DES for each curve. For each terrace we observe similar behavior for EBN: the mean EBN ranges from -519 mV to -534 mV, within one standard deviation of each other, suggesting relative uniformity from one terrace to another. However, within each terrace the distribution of EBN is significantly broader than the mV energy resolution. In contrast, E2DES has a much tighter distribution within each terrace, with standard deviations smaller than the energy resolution. However, the mean varies from -317 mV in Fig. 2d to -243 mV in Fig. 2f, representing an upshift of 123 to 197 mV. Other STM studies of hBN/Cu(111) systems have observed very different shifts in surface state energy7,34,37, with the shift sensitive to adsorption energy and local disorder, which vary across the sample at larger length scales. In Figure 3 we observe the spatial variation in spectral weight of EBN and E2DES for two different terraces. Figs. 3a and d shows the topograph acquired simultaneously with the spectral maps shown in Figs. 3b-c and 3e-f, respectively. These maps are constant energy slices near EBN and E2DES of the spectral maps used in Figs. 2d and f. The topographs were acquired at constant current with setpoint I = 200 pA and V = -700mV. We observe that for each map the spectral weight of the EBN peak varies much more than the E2DES peak, by nearly a factor of 10. The variation in spectral weight of E2DES is comparable to the variation in spectral weight at other energies (see Supplemental Movies 1 and 2). This suggests hBN is relatively transparent at low energies with transparency locally independent of the hBN morphology. The EBN peak not only varies in spectral weight considerably more across the map, but the variation is closely correlated with the Moire pattern, with the EBN peak much higher near the A sites of the Moire pattern. The peak energy EBN correlates with the Moire pattern as well, in addition to the intensity of the peak. Fig. 4c shows the dI/dV spectra from the map shown in Fig. 3d ordered by the location of EBN and color coded by distance to the Moire A sites. One can see that higher EBN (closer to the Fermi level) is correlated with spectra acquired closer to the Moire A sites. The opposite is true for the B sites, and no significant correlation is found between EBN and the C sites (see Supplemental Figure 1). In striking contrast, the location of E2DES (Fig. 4d) does not correlate with distance to the Moire A site (nor with B or C). In addition to being uncorrelated with the Moire pattern, the total range of E2DES is close to the error bars of the me
更多查看译文
AI 理解论文
溯源树
样例
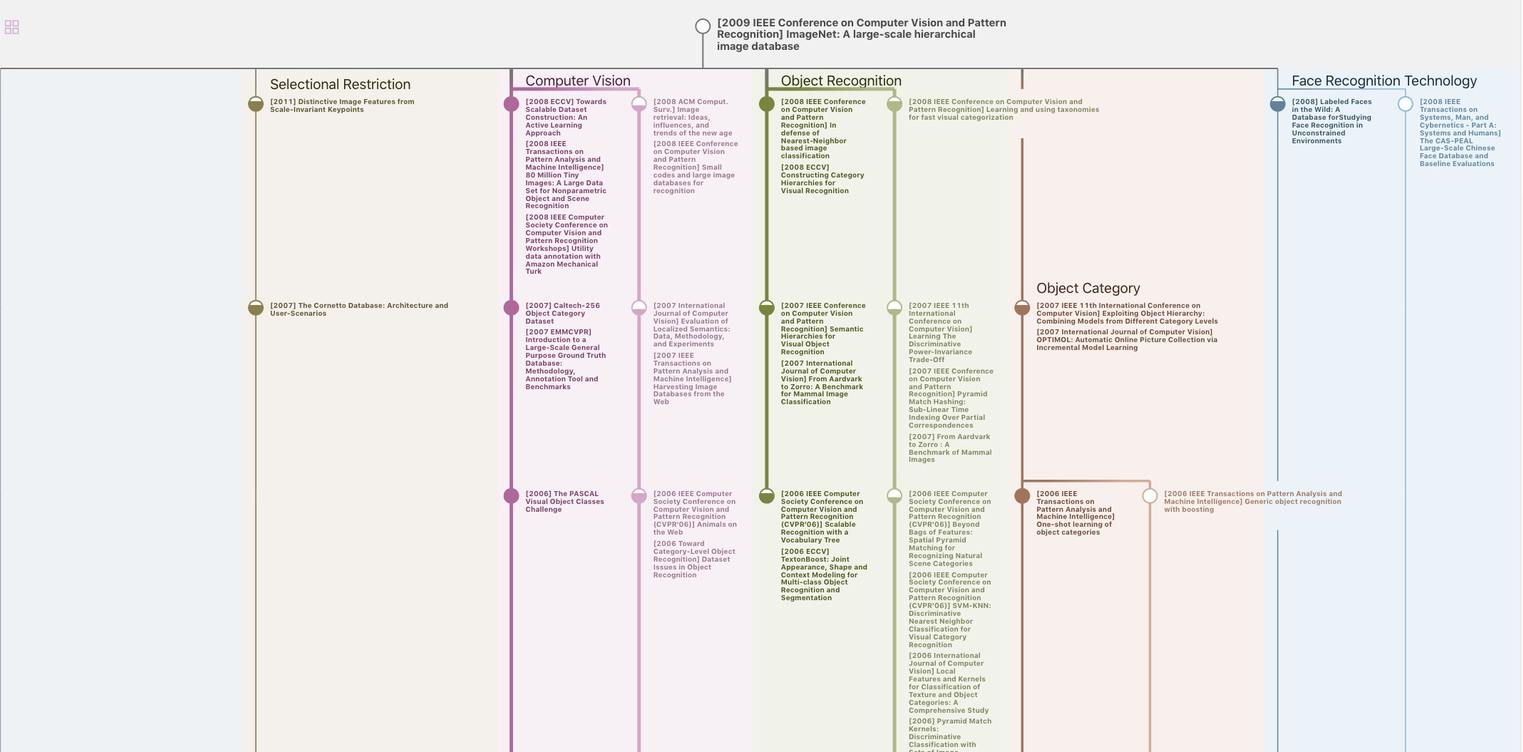
生成溯源树,研究论文发展脉络
Chat Paper
正在生成论文摘要