Supernumerary Limbs and Wearable Sensorimotor Interfaces
semanticscholar(2020)
摘要
The goal of this paper is to describe our studies on the Sixth Finger and present the different solutions available to control and to get haptic feedback from the system. The path toward the final solutions adopted has been paved with different intuitions about the designers and, more importantly, with continuous feedback from patients that tested all the proposed solutions a led us to the final setup. The paper is organised as follows. We will first recall the main characteristic of the Robotic Sixth Finger. Than we will go through the proposed solutions recalling the main results and pointing to the published papers for the details. I. THE ROBOTIC SIXTH FINGER A. Design of the Soft Sixth Finger The Soft-SixthFinger is a device designed to be used to compensate the missing grasping abilities of chronic stroke patients as presented in [1], [2]. The exploded view of the device and its possible applications are shown in Figure1. The working principle of the device is to replicate the two parts of a simple gripper using on one side the paretic forearm of a patient and, on the other side, a flexible finger that can be worn at the wrist with the help of an elastic band. The Soft-SixthFinger is built with a modular structure. Each module is composed of a rigid 3D printed part realized in ABS (Acrylonitrile Butadiene Styrene, ABSPlus, Stratasys, USA) and a 3D printed thermoplastic polyurethane part (Lulzbot, USA) that acts as the flexible joint. B. The Double Soft Sixth Finger Although the soft sixth finger can be used to grasp and stabilize a large set of objects, having a single finger in opposition to the patient arm can result in a limitation in tasks requiring a high payload. We designed the double soft sixth finger to deal with these particular situations [3]. The double soft sixth finger shares with the soft sixth finger the same principle design guidelines related to wearability, modularity, symmetrical structure and underactuation. It is composed of two parts: a support base that allows the finger to be worn at the patient forearm and two fingers. We fixed the fingers in a “V” configuration. The basic idea behind setting the two fingers in this configuration was to keep minimum distance at the base of the fingers while maximizing the fingertips’ distance at a fully extended position with the given length of 1Università degli Studi di Siena, Dipartimento di Ingegneria dell’Informazione, Via Roma 56, 53100 Siena, Italy. {prattichizzo}@dii.unisi.it 2Department of Advanced Robotics, Istituto Italiano di Tecnologia, Via Morego 30, 16163 Genoa, Italy. 3Department of Medicine, Surgery and Neuroscience, Unit of Neurology and Clinical Neurophysiology, Siena Brain Investigation and Neuromodulation Lab (Si-BIN Lab), University of Siena, Policlinico Le Scotte, Viale Bracci, I-53100 Siena, Italy Fig. 1: The Robotic Sixth Finger exploded view and its working principle with paretic hand to compensate its missing abilities. the finger. The rationale between this choice is the attempt to maximize the distance of the contact points at the fingertips when grasping relatively big size objects. Thanks to the orientation of the finger at the base, when the fingers keep closing so to grasp smaller size objects, the fingertips of both fingers converge one toward the other, hence minimizing the relative distance between them. Thus, this configuration is effective in grasping bigger as well as smaller size objects. The exploded view of the unit module and complete double soft sixth finger is shown in Figure 2. Two tendon wires (one for each flexible finger) and a single actuator control the motion of the device. One end of each tendon wire is fixed to each fingertip, while the other ends of both tendon wires are attached to a single pulley mounted on the shaft of the actuator (MX-28T). When the motor rotates, both tendon wires are wound on the pulley and fingers are flexed to grasp the object. As the motor is rotated in opposite direction, the elastic parts in the joints restore the finger to its extended configuration. The final prototype of the device is shown in Figure 3. the double soft sixth finger can be worn on the arm using the base support and the velcro strips. Both fingers can be shaped into a bracelet through two separate Dovetail locking mechanism when being not used. 2020 IEEE/RSJ International Conference on Intelligent Robots and Systems (IROS) October 25-29, 2020, Las Vegas, NV, USA (Virtual) 978-1-7281-6211-9/20/$31.00 ©2020 IEEE 12016 Fig. 2: The CAD exploded view of the double soft sixth finger. On the left unit module with single tendon. On the right, the exploded view of complete double soft sixth finger. Fig. 3: The final prototype of double soft sixth finger, the device can be worn on the paretic arm through support base and elastic straps. It can be shaped into bracelet when being not used.. II. EMG-BASED INTERFACE FOR THE ROBOTIC SIXTH FINGER The control interface for patient oriented devices must be intuitive and simple, since chronic patients may also be affected by some cognitive deficits, possibly limiting their compliance during a demanding learning phase. Coordinating the motion of the extra fingers with that of the hand where the devices are worn is not suitable for patients with hemiparetic upper limb since they are not able to control their hand motion. A possible solution could be the involvement of the controlateral hand in the control process. In [4], [5] a ring embedding a push-button to control the motion of an extra robotic finger was proposed. The ring was worn on the healthy hand so to let the user activate it when necessary. However, experiments with patients revealed that these solutions limit the mobility and dexterity of the nonparetic hand and can also cause possible accidental activation of the device during ADL. Patients also confirmed their preference of always having healthy hand free during our tests. To cope with this issue, we have proposed the eCap: an Electromyography (EMG) based wireless interface which maintains the principle of simplicity of a switch without interrupting the patient activities and without the involvement of a healthy hand during task execution. The eCap is a wearable wireless EMG interface where electrodes, acquisition and signal conditioning boards are embedded in a cap, see Figure 4 A preliminary version of the control interface has been presented in [2]. More details on the interface and on its possible applications can be found in [6], [3], [7], [8], [9]. Fig. 4: The eCap interface. The eCap allows the patients to autonomously wear the interface using only their healthy hand. For chronic patients it is generally difficult to generate repeatable EMG patterns in their paretic upper limb due to the weakness in muscle contraction control. For this reason, we coupled the flexion/extension motion of the robotic device with the contraction of the frontalis muscle. This muscle is always spared in case of a motor stroke either of the left or of the right hemisphere due to its bilateral cortical representation. The user can contract this muscle by moving the eyebrows upwards. The electrodes in the eCap capture the arising EMG signal that is acquired through an EMG signal conditioning circuit and processed by a control algorithm as explained in the following. We used surface EMG electrodes to measure electrical signals associated with the patient’s frontalis muscle. In particular, on the inner side of the eCap, we installed non-gelled reusable silver/silver-chloride electrodes, as they present the lowest noise interface and are recommended for biopotentials recording. We designed an EMG signal acquisition board taking into consideration the requirements associated with bandwidth, dynamic range and physiological principles. The motion of Sixth Finger is controlled by using a trigger signal based finite state machine The trigger signal is obtained by using a single-threshold value defined as the 50% of the maximum voluntary contraction (MVC), a level that was repeatable and sustainable for the subject without producing undue fatigue during the use of the device. We set a minimum time (20 ms) in which the EMG signal has to constantly stay over the threshold to generate the trigger signal to prevent false activation due to glitches or to
更多查看译文
AI 理解论文
溯源树
样例
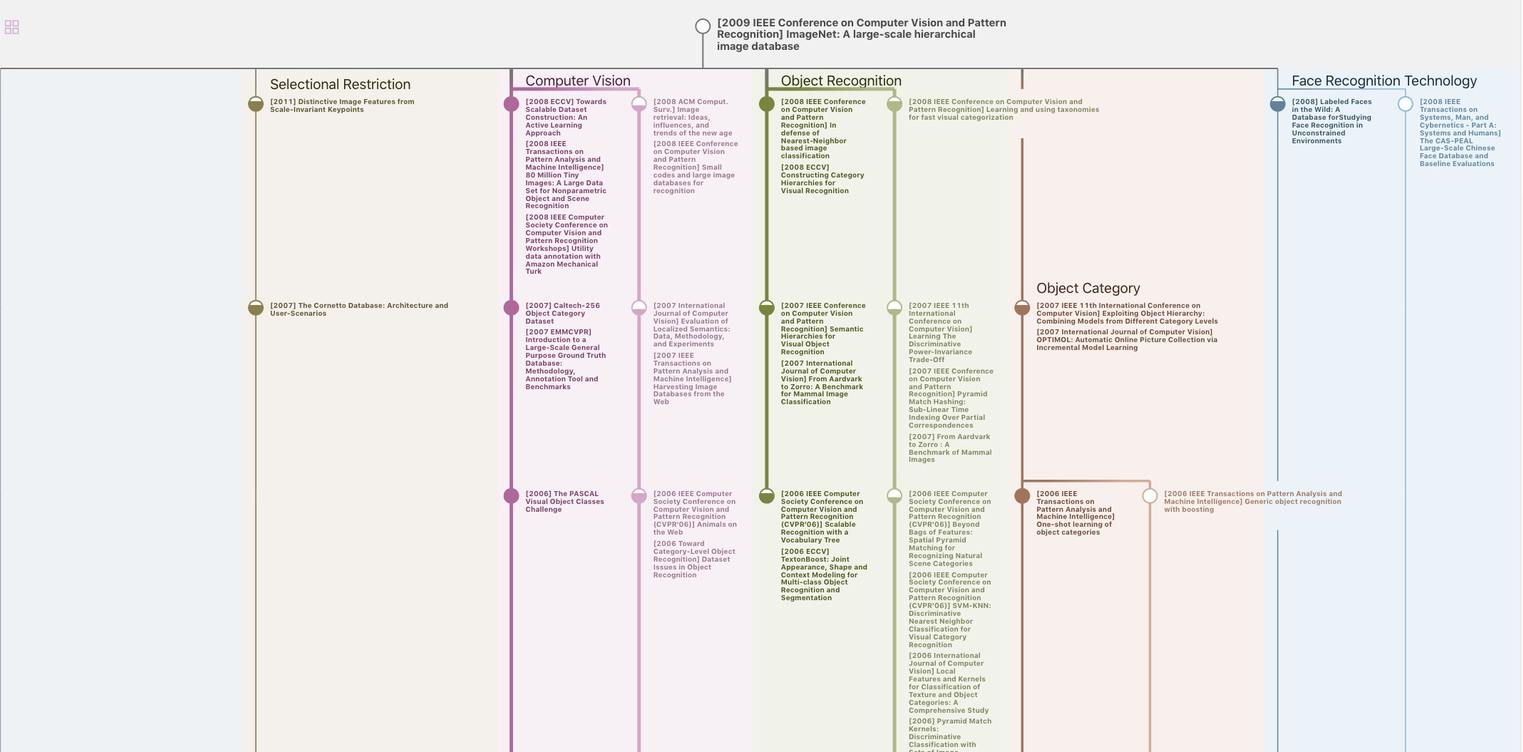
生成溯源树,研究论文发展脉络
Chat Paper
正在生成论文摘要