Nuclear Calcium Transients
Circulation(2014)
摘要
HomeCirculationVol. 130, No. 3Nuclear Calcium Transients Free AccessEditorialPDF/EPUBAboutView PDFView EPUBSections ToolsAdd to favoritesDownload citationsTrack citationsPermissions ShareShare onFacebookTwitterLinked InMendeleyReddit Jump toFree AccessEditorialPDF/EPUBNuclear Calcium TransientsHermes Propylaios in the Heart Paula A. da Costa Martins, PhD, Stefanos Leptidis, PhD and Leon J. De Windt, PhD Paula A. da Costa MartinsPaula A. da Costa Martins From the Department of Cardiology, School for Cardiovascular Diseases, Faculty of Health, Medicine, and Life Sciences, Maastricht University, Maastricht, The Netherlands. Search for more papers by this author , Stefanos LeptidisStefanos Leptidis From the Department of Cardiology, School for Cardiovascular Diseases, Faculty of Health, Medicine, and Life Sciences, Maastricht University, Maastricht, The Netherlands. Search for more papers by this author and Leon J. De WindtLeon J. De Windt From the Department of Cardiology, School for Cardiovascular Diseases, Faculty of Health, Medicine, and Life Sciences, Maastricht University, Maastricht, The Netherlands. Search for more papers by this author Originally published13 Jun 2014https://doi.org/10.1161/CIRCULATIONAHA.114.010675Circulation. 2014;130:221–223Other version(s) of this articleYou are viewing the most recent version of this article. Previous versions: July 15, 2014: Previous Version 1 O cunning guide, son of Maia, I fear that you might steal my lyre and my curved bow. For you hold the office from Zeus to establish deeds of exchange among men throughout the fertile earth. But if you would suffer to swear the great oath of the gods for me, either by nodding your head or by the mighty water of the Styx, you would accomplish everything pleasing to my own heart.—Homeric Hymnes1In Greek Mythology, Hermes (Greek: ρμ ς), son of Zeus and the pleiad Maia, was perhaps the most complex deity of transitions and boundaries, contemporarily simplified as a messenger god that moved freely between the higher worlds at Olympus, our world with human mortals, and the grim underworld of the deceased. As the second youngest of the Olympian gods and symbolizing Hermes’ function as divine messenger, he is often sculptured as a youngster with winged sandals and winged cap and holding his symbolic herald’s staff, the Greek kerykeion, consisting of 2 snakes wrapped around a winged staff. Hermes received multiple epithets including Propylaios, freely translated as “at the gateway,” reflecting a deeper philosophical significance of Hermes.Article see p 244As with all imaginary Greek deities, Hermes’ actions do not describe a uniformly positive character. Tales rather tell of a complex, 3-layered personality, ranging from a truly uplifting spirit, as would be expected from his divine roots, to a character with an innocent yet cunning nature, but at times he also displayed unexpected evil. With these 3 faces, Hermes likely symbolized how our Greek ancestors segmented the universe, consisting of a noble and divine upper world physically located at Mount Olympus; planet Earth with all its living creatures including mankind; and finally, the dark side of disease, suffering, and death, represented by the underworld.Cytoplasmic Calcium and Excitation-Contraction CouplingThe multifaceted roles that calcium plays in the heart may well bear analogy to the mythological character Hermes. First and foremost, for the maintenance of cardiac contraction, there may be no more important ion than calcium. During each heartbeat, a small leak of calcium through voltage-dependent L-type calcium channels triggers massive calcium release from the sarcoplasmatic reticulum (SR) through SR-bound ryanodine receptors (RyR2) into the cytoplasm of heart muscle cells, which triggers muscle contraction in a process referred to as excitation-contraction coupling.2 Cardiac relaxation is initiated by the concerted action of the sodium-calcium exchanger and muscle-specific SR calcium ATPase-2 (SERCA2), which resequesters calcium into the internal calcium storage pool, allowing for the next quantal release of calcium. In addition to this elaborate system of dedicated calcium release channels and calcium pumps, the β-adrenergic receptor system controls the magnitude of the calcium transient by activating protein kinase A, a kinase that by virtue of its direct phosphorylation of the calcium release channels and calcium pumps allows for intracellular calcium transients with higher amplitude and faster decay.Heart failure is characterized by disturbed excitation-contraction coupling and systolic and diastolic abnormal contractile properties, fueling speculations that correcting the calcium transient may form a rationale to recover cardiac contractility. Indeed, the cornerstone of contemporary heart failure pharmacotherapy is β-adrenergic blockade,3,4 and at least 1 rationale for its therapeutic benefit is suppression of the hyperadrenergic state and restored normal RyR2 calcium release channel function in patients with heart failure.5 In addition to that, at least 1 new therapeutic strategy seeking to normalize calcium transients in heart failure has now reached clinical stages in which viral-mediated gene therapy is directed to increase SERCA2a expression to improve SR Ca2+ reuptake.6Nucleoplasmic Calcium TransientsSecond, each cytoplasmic calcium transient also elicits nucleoplasmic calcium transients, which is a far less studied rhythmic phenomenon that also takes place simultaneously during cardiac contraction.7 Potentially, alterations in the kinetics or amplitude of nucleoplasmic calcium transients may have functional ramifications because this pool of calcium represents the prime candidate to influence calcium-dependent gene regulatory signaling events in a phenomenon referred to as excitation-transcription coupling.8 Indeed, a vast body of literature indicates that calcium/calmodulin acts as an important second messenger in the heart downstream of growth factors, adrenergic signaling, and mechanical stretch, which have the ability to trigger hypertrophic growth of the myocardium.9 Immediate recipient signals for calcium as second messenger are the calcium/calmodulin-dependent phosphatase calcineurin,10 which enables translocation of the transcription factor NFAT to the nucleus and in turn activates transcriptional pathways that reprogram cardiac gene expression either alone or by activating additional hypertrophic transcription factors including GATA4, MEF2, or Hand2.10,11 Alternatively, calcium/calmodulin-dependent protein kinase signaling relieves histone-deacetylase–dependent repression of MEF2 transcriptional activity and facilitates the induction of MEF2-responsive gene expression.12Nucleoplasmic calcium transients obey distinct boundaries, involve different partners, and play by slightly different rules than their cytoplasmic counterparts. First, the main store for nucleoplasmic calcium transients is formed by the nuclear envelope. As such, the nuclear envelope not only contributes to nuclear structure and insulation from the surrounding cytoplasm but also serves as the main reservoir for bidirectional transport of calcium ions and allows bidirectional transport of macromolecular complexes. The balance between release and storage of calcium in the nuclear envelope is regulated by calcium release channels, including the RyR2 and inositol 1,4,5-trisphosphate receptor, Ca2+-buffering proteins, and calcium pumps (SERCA2) that refill the nuclear envelope with calcium,13 reminiscent of the SR storage control.Prior characterizations of the nucleoplasmic calcium transient established that generation of the systolic calcium peak likely involves both a passive and an active component. The passive component is probably mediated by cytoplasmic calcium diffusion through nuclear pore complexes to increase nuclear calcium concentration because the amplitude of the nuclear calcium transient is proportional to the amplitude of the cytoplasmic calcium transient.14 However, several lines of evidence also indicate that the nucleus is capable of actively storing and releasing calcium ions because individually studied myocytes displayed prominently higher nucleoplasmic diastolic calcium concentration and higher nucleoplasmic systolic calcium peaks.14 This observation is in accord with the fact that endothelin-1 can induce an independent increase in nuclear calcium without changing cytosolic calcium transients and that this form of calcium release originates from perinuclear stores.15Stimulation of heart rate frequency increases systolic nuclear calcium in parallel with cytoplasmic systolic calcium, but diastolic nuclear calcium rises substantially higher than cytoplasmic counterparts, demonstrating a slower decay of the nucleoplasmic calcium transient and a much higher buildup of nuclear calcium when diastole is shortened.14 Whether this frequency-dependent physiological maneuver has implications for excitation-transcription coupling has remained unclear to date. In conclusion, prior studies support the idea that calcium release from the nuclear envelope at least in part plays an active role in nuclear calcium transient generation and feed speculation that nucleoplasmic transients may possibly alter and contribute to pathophysiological processes.Nucleoplasmic Calcium Transients and Excitation-Transcription CouplingIn this issue of Circulation, a novel report from Ljubojevic and Pieske and coworkers now adds valuable new information to the pathophysiology of nuclear calcium homeostasis.16 Ljubojevic et al used a cross-species and multidisciplinary approach to systematically compare the structural and functional changes of the nuclear envelope and nuclear calcium handling in myocytes derived from the healthy myocardium, the early hypertrophic phase, and late-stage heart failure.16 In short, early during hypertrophy, the authors observed an increased nuclear size and less frequent intrusions (invaginations) of the nuclear envelope into the nuclear lumen.Accompanying these ultrastructural changes, reduced expression of the calcium release channel RyR2 and the calcium pump SERCA2 versus an increase in inositol 1,4,5-trisphosphate receptor, as well as a differential localization of these various calcium transporters at the nuclear envelope, was observed. Most importantly, nuclear calcium transients displayed diminished amplitudes, lengthening of nuclear transient duration, and increased diastolic calcium levels, reminiscent of cytoplasmic calcium transients from failing myocytes.2 Finally, the ultrastructural changes of the envelope and alterations in nucleoplasmic transients were accompanied by a higher activation status of calcium/calmodulin-dependent protein kinase II and concomitant nuclear export of histone-deacetylase 4, suggesting a functional contribution of the altered nucleoplasmic transient in excitation-transcription coupling. These data therefore provide a plausible explanation for the mode of activation of calcium/calmodulin-induced gene regulatory systems in the earliest phases of heart failure.As with all breakthrough studies, these new observations also provoke a myriad of new questions that will stimulate the exploration of new experimental horizons. One less obvious question asks the rationale for the mere existence of nuclear transients in the healthy heart. It is likely that our lack of understanding this phenomenon must be related to a fine-tuned comprehension of the genomic architecture that may require calcium-dependent processes for normal nuclear function. Because similar findings have been observed in other organs besides the heart,17 it is expected that our field will witness the birth of new disciplines and technologies, including nuclear electrophysiology and optimized fluorescent reporter systems.18 Other questions are whether the tubular invaginations of the nuclear envelope resemble sarcolemmal T-tubuli, allowing juxtaposition of yet to be discovered critical calcium release channels and fine-tuned control of nuclear calcium, and what mechanism provokes the observed reduction of nuclear pore intrusions. On a more molecular level, readily testable hypotheses that now arise are whether the various calcium release channels and pumps also undergo posttranslational modifications similar to their counterparts at the sarcolemma and SR and, if so, at which amino acid sites does this occur, controlled by which balance of kinases/phosphatases? In addition, what functional ramifications may these biochemical changes provoke? A particularly intriguing aspect of the present study is the observation that with higher heart rate frequency, nuclear diastolic calcium concentration increased to a much higher extent by a slower decay than cytoplasmic transients.15 It is tempting to speculate that the frequency-dependent rise in diastolic nuclear calcium is causally related to the activation of prohypertrophic gene regulatory cascades and translates to the often elevated heart rates of patients with heart failure. Indeed, the Systolic Heart Failure Treatment With If Inhibitor Ivabradine Trial data demonstrate a beneficial effect of lowering heart rate by ivabradine in patients with advanced systolic heart failure in terms of both attenuated left ventricular remodeling and less frequent hospitalizations.19 To more firmly establish whether tachycardia is causally related to the earlier phases of cardiac remodeling, animal models of heart failure can be placed on heart rate–lowering medication with simultaneous monitoring of nuclear calcium transients, cardiac remodeling, and disease symptoms.Notwithstanding these as yet unanswered questions, the present study is in support of the premise that the nuclear ultrastructure is causally related to alterations in nuclear calcium transient generation and the pathophysiological phenomenon of excitation-transcription coupling. The multifaceted functions of the seemingly simple ion calcium transversing physical boundaries and communicating at the levels of the sarcomere, nuclear integrity, and excitation-transcription coupling are not unlike those of the mythological Hermes, influencing the divine sarcomeric contraction and homeostatic nuclear calcium transients in life and igniting myocardial disease and death.Sources of FundingDr da Costa Martins was supported by a Leducq Career Development Award and the Dutch Heart Foundation grant NHS2010B261. Dr De Windt acknowledges support from the Netherlands Cardiovascular Research Initiative, Dutch Heart Foundation, Dutch Federation of University Medical Centers, Netherlands Organization for Health Research and Development, and Royal Netherlands Academy of Sciences. Dr De Windt was further supported by the Fondation Leducq Transatlantic Network of Excellence program 08-CVD-03 and grant 311549 from the European Research Council.DisclosuresNone.FootnotesThe opinions expressed in this article are not necessarily those of the editors or of the American Heart Association.Correspondence to Leon J. De Windt, PhD, Department of Cardiology, School for Cardiovascular Diseases, Faculty of Health, Medicine, and Life Sciences, Maastricht University, Maastricht, Netherlands. E-mail [email protected]References1. Homer. Homeric Hymns to Hermes. IV-II, lines513–520.Google Scholar2. Bers DM. Cardiac excitation-contraction coupling.Nature. 2002; 415:198–205.CrossrefMedlineGoogle Scholar3. Poole-Wilson PA. The Cardiac Insufficiency Bisoprolol Study II.Lancet. 1999; 353:1360–1361.CrossrefMedlineGoogle Scholar4. Effect of metoprolol CR/XL in chronic heart failure: Metoprolol CR/XL Randomised Intervention Trial in Congestive Heart Failure (MERIT-HF).Lancet. 1999; 353:2001–2007.CrossrefMedlineGoogle Scholar5. Reiken S, Wehrens XH, Vest JA, Barbone A, Klotz S, Mancini D, Burkhoff D, Marks AR. Beta-blockers restore calcium release channel function and improve cardiac muscle performance in human heart failure.Circulation. 2003; 107:2459–2466.LinkGoogle Scholar6. Jessup M, Greenberg B, Mancini D, Cappola T, Pauly DF, Jaski B, Yaroshinsky A, Zsebo KM, Dittrich H, Hajjar RJ; Calcium Upregulation by Percutaneous Administration of Gene Therapy in Cardiac Disease (CUPID) Investigators. Calcium Upregulation by Percutaneous Administration of Gene Therapy in Cardiac Disease (CUPID): a phase 2 trial of intracoronary gene therapy of sarcoplasmic reticulum Ca2+-ATPase in patients with advanced heart failure.Circulation. 2011; 124:304–313.LinkGoogle Scholar7. Yang Z, Steele DS. Characteristics of prolonged Ca2+ release events associated with the nuclei in adult cardiac myocytes.Circ Res. 2005; 96:82–90.LinkGoogle Scholar8. Maier LS, Bers DM. Calcium, calmodulin, and calcium-calmodulin kinase II: heartbeat to heartbeat and beyond.J Mol Cell Cardiol. 2002; 34:919–939.CrossrefMedlineGoogle Scholar9. Frey N, McKinsey TA, Olson EN. Decoding calcium signals involved in cardiac growth and function.Nat Med. 2000; 6:1221–1227.CrossrefMedlineGoogle Scholar10. Molkentin JD, Lu JR, Antos CL, Markham B, Richardson J, Robbins J, Grant SR, Olson EN. A calcineurin-dependent transcriptional pathway for cardiac hypertrophy.Cell. 1998; 93:215–228.CrossrefMedlineGoogle Scholar11. Dirkx E, Gladka MM, Philippen LE, Armand AS, Kinet V, Leptidis S, El Azzouzi H, Salic K, Bourajjaj M, da Silva GJ, Olieslagers S, van der Nagel R, de Weger R, Bitsch N, Kisters N, Seyen S, Morikawa Y, Chanoine C, Heymans S, Volders PG, Thum T, Dimmeler S, Cserjesi P, Eschenhagen T, da Costa Martins PA, De Windt LJ. Nfat and miR-25 cooperate to reactivate the transcription factor Hand2 in heart failure.Nat Cell Biol. 2013; 15:1282–1293.CrossrefMedlineGoogle Scholar12. Zhang CL, McKinsey TA, Chang S, Antos CL, Hill JA, Olson EN. Class II histone deacetylases act as signal-responsive repressors of cardiac hypertrophy.Cell. 2002; 110:479–488.CrossrefMedlineGoogle Scholar13. Echevarría W, Leite MF, Guerra MT, Zipfel WR, Nathanson MH. Regulation of calcium signals in the nucleus by a nucleoplasmic reticulum.Nat Cell Biol. 2003; 5:440–446.CrossrefMedlineGoogle Scholar14. Ljubojević S, Walther S, Asgarzoei M, Sedej S, Pieske B, Kockskämper J. In situ calibration of nucleoplasmic versus cytoplasmic Ca²+ concentration in adult cardiomyocytes.Biophys J. 2011; 100:2356–2366.CrossrefMedlineGoogle Scholar15. Kockskämper J, Seidlmayer L, Walther S, Hellenkamp K, Maier LS, Pieske B. Endothelin-1 enhances nuclear Ca2+ transients in atrial myocytes through Ins(1,4,5)P3-dependent Ca2+ release from perinuclear Ca2+ stores.J Cell Sci. 2008; 121(pt 2):186–195.CrossrefMedlineGoogle Scholar16. Ljubojevic S, Radulovic S, Leitinger G, Sedej S, Sacherer M, Holzer M, Winkler C, Pritz E, Mittler T, Schmidt A, Sereinigg M, Wakula P, Zissimopoulos S, Bisping E, Post H, Marsche G, Bossuyt J, Bers DM, Kockskamper J, Pieske B. Early remodelling of perinuclear Ca2+ stores and nucleoplasmic Ca2+ signaling during the development of hypertrophy and heart failure.Circulation. 2014; 130:244–255.LinkGoogle Scholar17. Quesada I, Rovira JM, Martin F, Roche E, Nadal A, Soria B. Nuclear KATP channels trigger nuclear Ca(2+) transients that modulate nuclear function.Proc Natl Acad Sci U S A. 2002; 99:9544–9549.CrossrefMedlineGoogle Scholar18. Matzke AJ, Weiger TM, Matzke M. Ion channels at the nucleus: electrophysiology meets the genome.Mol Plant. 2010; 3:642–652.CrossrefMedlineGoogle Scholar19. Tardif JC, O’Meara E, Komajda M, Böhm M, Borer JS, Ford I, Tavazzi L, Swedberg K; SHIFT Investigators. Effects of selective heart rate reduction with ivabradine on left ventricular remodelling and function: results from the SHIFT echocardiography substudy.Eur Heart J. 2011; 32:2507–2515.CrossrefMedlineGoogle Scholar Previous Back to top Next FiguresReferencesRelatedDetailsCited ByQi X, Vahdati Hassani F, Hoffmann D, Xiao J, Xiong F, Villeneuve L, Ljubojevic-Holzer S, Kamler M, Abu-Taha I, Heijman J, Bers D, Dobrev D and Nattel S (2020) Inositol Trisphosphate Receptors and Nuclear Calcium in Atrial Fibrillation, Circulation Research, 128:5, (619-635), Online publication date: 5-Mar-2021. July 15, 2014Vol 130, Issue 3 Advertisement Article InformationMetrics © 2014 American Heart Association, Inc.https://doi.org/10.1161/CIRCULATIONAHA.114.010675PMID: 24928679 Originally publishedJune 13, 2014 KeywordsEditorialsPDF download Advertisement SubjectsAnimal Models of Human Disease
更多查看译文
关键词
Calcium Channels,Calcium Signaling,Cardiac Electrophysiology,Cardiac Imaging
AI 理解论文
溯源树
样例
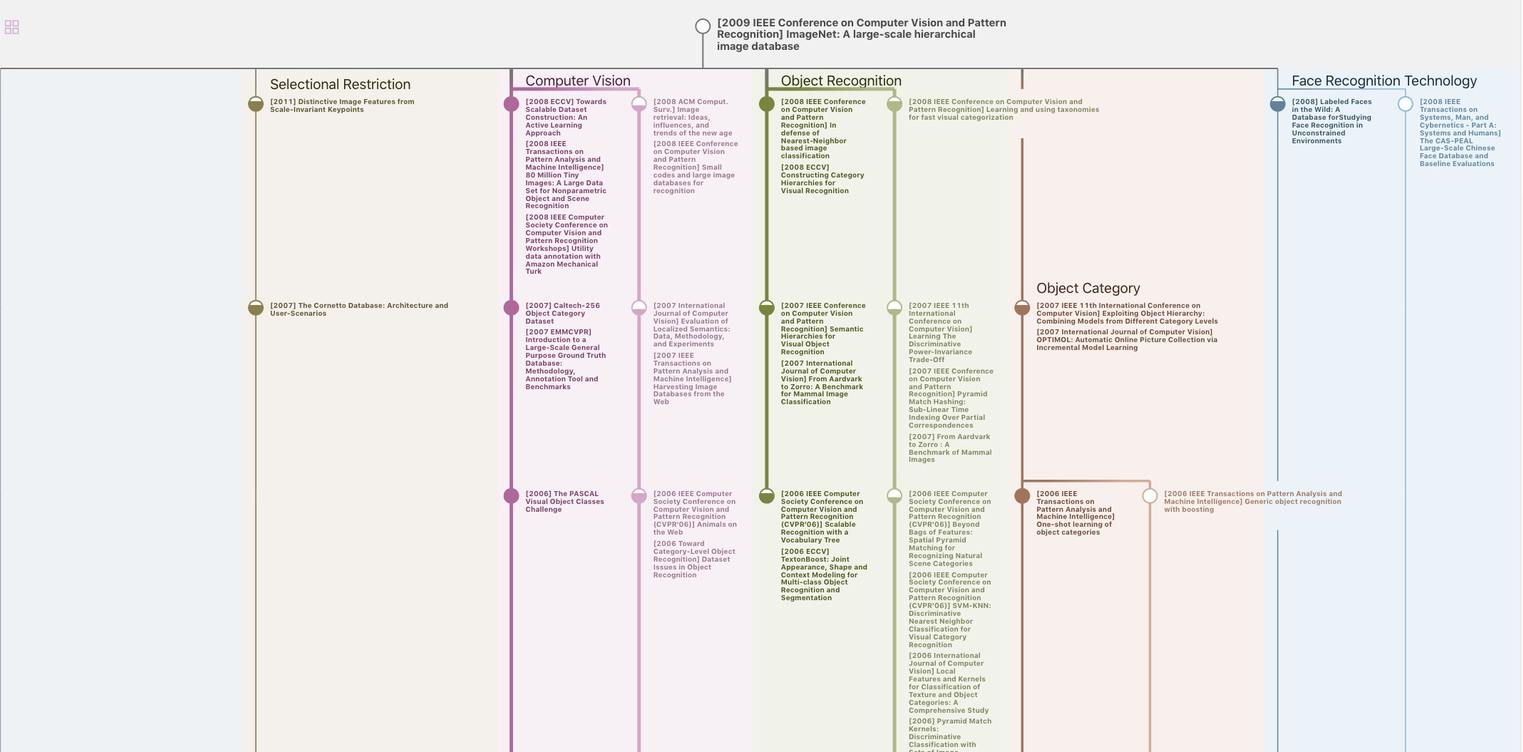
生成溯源树,研究论文发展脉络
Chat Paper
正在生成论文摘要