Synthetic lethality between
VPS
4A
and
VPS
4B
triggers an inflammatory response in colorectal cancer
EMBO Molecular Medicine(2020)
摘要
Article13 January 2020Open Access Source DataTransparent process Synthetic lethality between VPS4A and VPS4B triggers an inflammatory response in colorectal cancer Ewelina Szymańska Corresponding Author Ewelina Szymańska [email protected] orcid.org/0000-0001-7994-0764 Laboratory of Cell Biology, International Institute of Molecular and Cell Biology, Warsaw, Poland Search for more papers by this author Paulina Nowak Paulina Nowak Laboratory of Cell Biology, International Institute of Molecular and Cell Biology, Warsaw, Poland Search for more papers by this author Krzysztof Kolmus Krzysztof Kolmus Laboratory of Cell Biology, International Institute of Molecular and Cell Biology, Warsaw, Poland Search for more papers by this author Magdalena Cybulska Magdalena Cybulska Department of Genetics, Maria Skłodowska-Curie Institute-Oncology Centre, Warsaw, Poland Search for more papers by this author Krzysztof Goryca Krzysztof Goryca Department of Genetics, Maria Skłodowska-Curie Institute-Oncology Centre, Warsaw, Poland Search for more papers by this author Edyta Derezińska-Wołek Edyta Derezińska-Wołek Department of Pathology and Laboratory Medicine, Maria Skłodowska-Curie Institute-Oncology Centre, Warsaw, Poland Department of Diagnostic Hematology, Institute of Hematology and Transfusion Medicine, Warsaw, Poland Search for more papers by this author Anna Szumera-Ciećkiewicz Anna Szumera-Ciećkiewicz Department of Pathology and Laboratory Medicine, Maria Skłodowska-Curie Institute-Oncology Centre, Warsaw, Poland Department of Diagnostic Hematology, Institute of Hematology and Transfusion Medicine, Warsaw, Poland Search for more papers by this author Marta Brewińska-Olchowik Marta Brewińska-Olchowik Laboratory of Cytometry, Nencki Institute of Experimental Biology, Warsaw, Poland Search for more papers by this author Aleksandra Grochowska Aleksandra Grochowska Department of Genetics, Maria Skłodowska-Curie Institute-Oncology Centre, Warsaw, Poland Department of Gastroenterology, Hepatology and Clinical Oncology, Medical Center for Postgraduate Education, Warsaw, Poland Search for more papers by this author Katarzyna Piwocka Katarzyna Piwocka Laboratory of Cytometry, Nencki Institute of Experimental Biology, Warsaw, Poland Search for more papers by this author Monika Prochorec-Sobieszek Monika Prochorec-Sobieszek Department of Pathology and Laboratory Medicine, Maria Skłodowska-Curie Institute-Oncology Centre, Warsaw, Poland Department of Diagnostic Hematology, Institute of Hematology and Transfusion Medicine, Warsaw, Poland Search for more papers by this author Michał Mikula Michał Mikula Department of Genetics, Maria Skłodowska-Curie Institute-Oncology Centre, Warsaw, Poland Search for more papers by this author Marta Miączyńska Corresponding Author Marta Miączyńska [email protected] orcid.org/0000-0003-0031-5267 Laboratory of Cell Biology, International Institute of Molecular and Cell Biology, Warsaw, Poland Search for more papers by this author Ewelina Szymańska Corresponding Author Ewelina Szymańska [email protected] orcid.org/0000-0001-7994-0764 Laboratory of Cell Biology, International Institute of Molecular and Cell Biology, Warsaw, Poland Search for more papers by this author Paulina Nowak Paulina Nowak Laboratory of Cell Biology, International Institute of Molecular and Cell Biology, Warsaw, Poland Search for more papers by this author Krzysztof Kolmus Krzysztof Kolmus Laboratory of Cell Biology, International Institute of Molecular and Cell Biology, Warsaw, Poland Search for more papers by this author Magdalena Cybulska Magdalena Cybulska Department of Genetics, Maria Skłodowska-Curie Institute-Oncology Centre, Warsaw, Poland Search for more papers by this author Krzysztof Goryca Krzysztof Goryca Department of Genetics, Maria Skłodowska-Curie Institute-Oncology Centre, Warsaw, Poland Search for more papers by this author Edyta Derezińska-Wołek Edyta Derezińska-Wołek Department of Pathology and Laboratory Medicine, Maria Skłodowska-Curie Institute-Oncology Centre, Warsaw, Poland Department of Diagnostic Hematology, Institute of Hematology and Transfusion Medicine, Warsaw, Poland Search for more papers by this author Anna Szumera-Ciećkiewicz Anna Szumera-Ciećkiewicz Department of Pathology and Laboratory Medicine, Maria Skłodowska-Curie Institute-Oncology Centre, Warsaw, Poland Department of Diagnostic Hematology, Institute of Hematology and Transfusion Medicine, Warsaw, Poland Search for more papers by this author Marta Brewińska-Olchowik Marta Brewińska-Olchowik Laboratory of Cytometry, Nencki Institute of Experimental Biology, Warsaw, Poland Search for more papers by this author Aleksandra Grochowska Aleksandra Grochowska Department of Genetics, Maria Skłodowska-Curie Institute-Oncology Centre, Warsaw, Poland Department of Gastroenterology, Hepatology and Clinical Oncology, Medical Center for Postgraduate Education, Warsaw, Poland Search for more papers by this author Katarzyna Piwocka Katarzyna Piwocka Laboratory of Cytometry, Nencki Institute of Experimental Biology, Warsaw, Poland Search for more papers by this author Monika Prochorec-Sobieszek Monika Prochorec-Sobieszek Department of Pathology and Laboratory Medicine, Maria Skłodowska-Curie Institute-Oncology Centre, Warsaw, Poland Department of Diagnostic Hematology, Institute of Hematology and Transfusion Medicine, Warsaw, Poland Search for more papers by this author Michał Mikula Michał Mikula Department of Genetics, Maria Skłodowska-Curie Institute-Oncology Centre, Warsaw, Poland Search for more papers by this author Marta Miączyńska Corresponding Author Marta Miączyńska [email protected] orcid.org/0000-0003-0031-5267 Laboratory of Cell Biology, International Institute of Molecular and Cell Biology, Warsaw, Poland Search for more papers by this author Author Information Ewelina Szymańska *,1, Paulina Nowak1, Krzysztof Kolmus1, Magdalena Cybulska2, Krzysztof Goryca2, Edyta Derezińska-Wołek3,4, Anna Szumera-Ciećkiewicz3,4, Marta Brewińska-Olchowik5, Aleksandra Grochowska2,6, Katarzyna Piwocka5, Monika Prochorec-Sobieszek3,4, Michał Mikula2 and Marta Miączyńska *,1 1Laboratory of Cell Biology, International Institute of Molecular and Cell Biology, Warsaw, Poland 2Department of Genetics, Maria Skłodowska-Curie Institute-Oncology Centre, Warsaw, Poland 3Department of Pathology and Laboratory Medicine, Maria Skłodowska-Curie Institute-Oncology Centre, Warsaw, Poland 4Department of Diagnostic Hematology, Institute of Hematology and Transfusion Medicine, Warsaw, Poland 5Laboratory of Cytometry, Nencki Institute of Experimental Biology, Warsaw, Poland 6Department of Gastroenterology, Hepatology and Clinical Oncology, Medical Center for Postgraduate Education, Warsaw, Poland *Corresponding author. Tel: +48 22 597 07 27; E-mail: [email protected] *Corresponding author. Tel: +48 22 597 07 25; E-mail: [email protected] EMBO Mol Med (2020)12:e10812https://doi.org/10.15252/emmm.201910812 PDFDownload PDF of article text and main figures. Peer ReviewDownload a summary of the editorial decision process including editorial decision letters, reviewer comments and author responses to feedback. ToolsAdd to favoritesDownload CitationsTrack CitationsPermissions ShareFacebookTwitterLinked InMendeleyWechatReddit Figures & Info Abstract Somatic copy number alterations play a critical role in oncogenesis. Loss of chromosomal regions containing tumor suppressors can lead to collateral deletion of passenger genes. This can be exploited therapeutically if synthetic lethal partners of such passenger genes are known and represent druggable targets. Here, we report that VPS4B gene, encoding an ATPase involved in ESCRT-dependent membrane remodeling, is such a passenger gene frequently deleted in many cancer types, notably in colorectal cancer (CRC). We observed downregulation of VPS4B mRNA and protein levels from CRC patient samples. We identified VPS4A paralog as a synthetic lethal interactor for VPS4B in vitro and in mouse xenografts. Depleting both proteins profoundly altered the cellular transcriptome and induced cell death accompanied by the release of immunomodulatory molecules that mediate inflammatory and anti-tumor responses. Our results identify a pair of novel druggable targets for personalized oncology and provide a rationale to develop VPS4 inhibitors for precision therapy of VPS4B-deficient cancers. Synopsis VPS4B and VPS4A paralogs are involved in the remodeling of biological membranes, a critical step for many intracellular processes. This study highlights the possibility of using synthetic lethality between these paralogs for treatment of VPS4B-deficient cancers. VPS4B protein abundance was decreased in colorectal cancer (CRC) patient samples. A synthetic lethal phenotype was generated by simultaneous depletion of VPS4A and B in various CRC cell lines grown in vitro and in vivo. Synthetic lethality between VPS4A and B was independent of other oncogenic mutations, and conserved between human and mouse, thus of high penetrance. Simultaneous depletion of VPS4A and B caused pleiotropic effects e.g. inhibited endocytosis and cell cycle progression, and induced a stress-associated sterile inflammatory response. DAMPs and other immunomodulatory molecules released by VPS4A+B-depleted dying cells may favor the induction of anti-tumor innate and adaptive immune responses. Introduction Somatic copy number alterations are a key driving force in malignant transformation (Beroukhim et al, 2010). In particular, chromosomal deletions may affect 35% of the cancer genome (Beroukhim et al, 2010). Large deletions may include loss of a tumor suppressor locus, correlating with concomitant loss of hundreds of neighboring protein-coding genes and other regulatory elements. However, it remains elusive whether and how these passenger alterations contribute to cancer development and progression. Nevertheless, passenger alterations may confer a weakness to cancer cells that could be exploited in cancer therapy using synthetic lethality-based approaches. A synthetic lethal interaction between two genes occurs when perturbation of either gene alone does not alter cell fitness but concomitant perturbation of both genes induces a lethal phenotype. This simple genetic concept underlies well-established studies that characterize functional interactions between two or more genes. Cancer research now exploits this principle to develop new genotype-specific anti-cancer therapeutics (O'Neil et al, 2017). Using this approach, PARP inhibitors were introduced as a treatment option for patients with BRCA1/2-mutated tumors (Lord & Ashworth, 2017). Continued efforts to develop the synthetic lethality concept for cancer research focus on targeting a non-oncogenic alteration by identifying its second-site synthetically lethal interactor gene. But, developing anti-cancer therapies based on this concept urgently requires identifying and characterizing novel synthetic lethal partners. Chromosome 18 carries both known and candidate tumor suppressor genes like the well-characterized DCC [Deleted in Colon Cancer Gene], SMAD2, and SMAD4 (Nguyen & Duong, 2018). Loss of heterozygosity (LOH) at the long arm of this chromosome (18q) can occur in colon (Ogunbiyi et al, 1998; Sheffer et al, 2009), pancreatic (Sunamura et al, 2004), lung (Takei et al, 1998), prostate (Kluth et al, 2016), and breast cancers (Huiping et al, 1998), as well as head and neck squamous cell carcinoma (Takebayashi et al, 2000). One gene localized on 18q is VPS4B (Vacuolar Protein Sorting 4 Homolog B). Its protein product VPS4B is a ubiquitously expressed type I AAA+ (ATPases Associated with diverse cellular Activities) ATPase (Han & Hill, 2019). It cooperates with its highly homologous paralog VPS4A (encoded by the VPS4A gene on 16q) to disassemble and release the Endosomal Sorting Complex Required for Transport (ESCRT) machinery from intracellular membranes, which enables recycling of ESCRT subunits (Henne et al, 2013; Schoneberg et al, 2017; McCullough et al, 2018). Endosomal Sorting Complex Required for Transport complexes drive membrane budding and scission at various intracellular compartments (Hurley, 2015) and contribute to a plethora of essential cellular processes, such as endocytic cargo sorting, autophagy, cytokinesis, exovesicle release, and repair of the nuclear envelope, the plasma membrane, and lysosomal membranes (reviewed in refs. Olmos & Carlton, 2016; Christ et al, 2017; Szymanska et al, 2018). In contrast to the well-established roles of ESCRT components in cell biology, their contribution to tumorigenesis, if any, is less documented (Mattissek & Teis, 2014; Alfred & Vaccari, 2016; Gingras et al, 2017) and the underlying molecular mechanisms have been elucidated for only a few cases (Manteghi et al, 2016; Sadler et al, 2018). A large-scale screening for cancer vulnerabilities within the Sanger's Project Score (Behan et al, 2019) and the DRIVE project (McDonald et al, 2017) revealed that some cancer cell lines are very sensitive to perturbed VPS4A expression. The authors of the latter report suggested the existence of a synthetic lethality between VPS4A and VPS4B; however, this hypothesis has not been experimentally verified. Here, we investigated whether VPS4B expression is perturbed in cancer samples and whether VPS4A is a synthetic lethal partner for VPS4B. Our findings reveal a novel druggable target for further translational research toward personalized therapies for colorectal cancer (CRC) patients. Results VPS4B expression is deregulated in multiple cancer types, prominently in CRC To study the extent of genetic changes at the VPS4B locus in different types of cancer, we mined The Cancer Genome Atlas (TCGA). The overview of Pan-Cancer TCGA somatic copy number alteration dataset revealed about a 30% incidence of chromosome 18q LOH at the VPS4B locus (Fig 1A). Further analysis of individual cancer datasets showed frequent VPS4B deletion in several types of cancer with CRC being the most affected (Fig 1B). In line with previous reports on 18q LOH in CRC (Sheffer et al, 2009), an overall incidence of the VPS4B loss in the TCGA CRC dataset was ~70% with bi-allelic deletion estimated at 2% (Fig 1C). In addition, the DNA copy number and mRNA levels of VPS4B were significantly correlated with a Pearson coefficient of 0.75. We next validated changes in VPS4B mRNA abundance using an independent set of CRC samples from our previous studies (Skrzypczak et al, 2010; Mikula et al, 2011). Using qRT–PCR analysis, we confirmed significantly downregulated VPS4B expression, which indicated that its mRNA levels decreased during progression from adenoma to adenocarcinoma (Fig 1D). In contrast, in the same samples, we detected no change in the level of VPS4A mRNA between normal colon, adenoma, and CRC (Fig EV1A). Figure 1. Expression of VPS4B is downregulated in CRC A. Left panel, a scheme of chromosome 18 copy number alterations depicting the distal long arm loss across TCGA Pan-Cancer dataset. Vertical red line indicates the localization of VPS4B. Right panel, enlarged fragment of chromosome 18 showing frequent deletions of VPSB locus in cancer samples. Deletions are marked in blue, and amplified regions are marked in red. Both panels were generated with UCSC Xena browser (https://www.biorxiv.org/content/10.1101/326470v3). B. Analysis of VPS4B copy number alterations in TCGA Pan-Cancer dataset. Cancer types were sorted according to the mean VPS4B copy number after removing germline values. The boxes denote the 25th to 75th percentile range, and the center lines mark the 50th percentile (median). The whiskers reflect the largest and smallest observed values. VPS4B copy number alteration data were fetched using UCSC Xena browser. C. Scatter plot, analysis of VPS4B mRNA expression (number of transcripts per million) plotted against VPS4B copy number from TCGA CRC patient samples (n = 376); plot generated using cBioPortal (Gao et al, 2013). Pie chart, summary of all types of VPS4B copy number alterations based on the analysis of data from 615 CRC samples deposited in the Colorectal Adenocarcinoma (TCGA, Provisional) dataset on cBioPortal (http://www.cbioportal.org/). D. qPCR analysis of VPS4B mRNA abundance in normal colon, adenoma, and CRC samples. Adenocarcinoma (n = 26); adenoma (n = 42); normal colon (n = 24). Green horizontal bars indicate means, and red whiskers indicate SD. Differences were analyzed using the Kruskal–Wallis test followed by Dunn's multiple comparison post hoc test; ns—non-significant (P ≥ 0.05), ****P < 0.0001. E. Examples of immunohistochemical staining of VPS4B in normal colon and matched CRC samples as an illustration of the scoring system used for the evaluation presented in (F). 3+—very intensive staining, 2+—medium-intensive staining, 1+—weak staining, 0—no staining. Scale bar, 50 μm. F. Comparative analysis of VPS4B staining performed in 100 pairs of normal colon and matched CRC samples. Data information: The exact P-values can be found in the source data for this figure. Source data are available online for this figure. Source Data for Figure 1 [emmm201910812-sup-0006-SDataFig1.xlsx] Download figure Download PowerPoint Click here to expand this figure. Figure EV1. Evaluation of VPS4A and VPS4B protein abundance in human tissues and in CRC A. qPCR analysis of VPS4A mRNA abundance in normal colon, adenoma, and CRC samples. Adenocarcinoma (n = 26); adenoma (n = 42); normal colon (n = 24). Green horizontal bars indicate means, and red whiskers indicate SD. Differences were analyzed using the Kruskal–Wallis test followed by Dunn's multiple comparison post hoc test; ns—non-significant (P ≥ 0.05). B. Immunohistochemistry (IHC) evaluation of VPS4A abundance in pairs of normal colon versus matched CRC samples (2 representative pairs out of 100 analyzed). 3+—very intensive staining. Scale bar, 20 μm. C. Specificity tests of VPS4B IHC staining using human tissues and mouse xenografts. C1—strong granular cytoplasmic staining in the mucosa of the appendix. C2, C3—negative staining in the appendix (C2) and CRC (C3) when primary antibody (anti-VPS4B) was omitted. C4—granular cytoplasmic staining in the xenograft from wild-type (VPS4B+/+) HCT116 cells. C5—negative staining in the xenograft from HCT116 VPS4B−/− cells. Scale bars: C1, C4, and C5—20 μm; C2, C3—50 μm. D. Specificity tests of VPS4A IHC staining in various human tissues with high and low expression of VPS4A. D1—strong granular cytoplasmic staining in the mucosa of the appendix (arrowhead, and at a higher magnification in D2) and weak staining in the muscle (arrow, and at a higher magnification in D3). Scale bars: D1—200 μm; D2 and D3—20 μm. Data information: The exact P-values can be found in Appendix Table S3. Download figure Download PowerPoint To examine whether genetic alterations at the VPS4B locus corresponded to decreased VPS4B protein abundance in CRC, we performed immunohistochemistry (IHC) staining of both paralogs of VPS4 in tissue microarrays covering one hundred pairs of matched human normal colon and treatment-naïve primary CRC samples (Figs 1E and F, and EV1B). We evaluated the microarrays using a semi-quantitative scoring method based on staining intensity (Fig 1E). Antibodies used for IHC staining had been previously tested and approved in The Human Protein Atlas project (https://www.proteinatlas.org/). We confirmed the specificity of the selected antibodies by staining normal human tissues with known high and low protein abundance of both VPS4 paralogs (appendix and muscle, respectively) (Fig EV1C and D). As an additional validation of anti-VPS4B antibody, we confirmed lack of VPS4B staining in mouse xenografts derived from HCT116 human CRC line with both VPS4B alleles inactivated by the CRISPR/Cas9 method (Fig EV1C4 and C5). When analyzing the tissue microarrays, we found that the staining of VPS4B protein from analyzed pairs of matched cancer patient samples was not as intense as in normal colon (Fig 1F, 3+→3+). In most cases (57%), we observed a slightly decreased VPS4B staining in cancer samples (very intense in normal colon versus medium intense in CRC; 3+→2+). However, we observed a much more prominent decrease of VPS4B staining in CRC (3+→1+) in 39% of tissue pairs and did not detect VPS4B protein in 4% of CRC of tissue samples (3+→0). In contrast, VPS4A staining using the same collection of patient samples demonstrated equally high VPS4A protein abundance in all normal colon and CRC matched pairs (Fig EV1B). Altogether, our analysis of TCGA databases revealed that the VPS4B allele is frequently lost in many cancer types, prominently in CRC. This genetic alteration correlates with decreased VPS4B mRNA and protein content in cancer tissues that we demonstrated using our collections of CRC patient samples. VPS4A is a synthetic lethal partner for VPS4B VPS4A and VPS4B are the only enzymes among the ESCRT subunits and represent a bottleneck for ESCRT-mediated processes, as no other known ATPases can substitute for their activity. Given our observed aberrations of the VPS4B locus in CRC, we hypothesized that VPS4B and its paralog VPS4A were synthetic lethal interactors. Initially, we chose an in vitro system of a near diploid HCT116 cell line, a human epithelial colorectal carcinoma in which the copy number of VPS4 paralogs is not altered according to the Cancer Cell Line Encyclopedia dataset (CCLE, https://portals.broadinstitute.org/ccle). We assessed its viability upon single RNAi-mediated depletion of VPS4A or VPS4B, as well as simultaneous depletion of VPS4A and VPS4B (referred to as VPS4A+B). We verified high knockdown efficiency and specificity of siRNAs (two or three independent sequences per target) by measuring mRNA and protein abundance of both paralogs (Fig EV2A and B). Despite the sequence similarity between VPS4A and VPS4B, we could efficiently silence the expression of a single VPS4A or VPS4B paralog or both. We revealed that loss of expression of one paralog did not affect the mRNA or protein level of the other (Fig EV2A and B). This suggests that cells with compromised expression of one VPS4 paralog possess no compensatory mechanisms and show no cross-destabilization of the remaining paralog. These results, together with the data showing no correlation between VPS4A and VPS4B mRNA and protein levels in patient samples (Figs 1D and EV1A; Figs 1F and EV1B, respectively), indicate that the regulation of gene expression and protein stability of both paralogs occurs independently. Click here to expand this figure. Figure EV2. Analyses of VPS4A and VPS4B mRNA and protein abundance in HCT116 cells and the dependencies between both genes in different cell lines A. mRNA levels of VPS4A (upper panel) and VPS4B (lower panel) in HCT116 cells 72 h upon siRNA transfection. For VPS4A or VPS4B depletion, two different duplexes (#1 or #2) of siVPS4A or siVPS4B were used. For simultaneous VPS4A+B depletion, various combinations of siVPS4A and siVPS4B duplexes were used. Two different duplexes of siCTRL (#1 or #2) were used as non-targeting controls. NT—non-transfected. Values represent normalized counts after including variance normalized transformation performed by the DESeq2 package for RNA-Seq data analysis. Data are means of four independent experiments ± SEM. Two-tailed unpaired t-test; ns—non-significant (P ≥ 0.05). B. Upper panel, representative immunoblotting analysis of VPS4A and VPS4B abundance in lysates of HCT116 cells collected 72 h after transfection as in (A). GAPDH was used as a loading control. Lower panel, densitometry analysis of VPS4A and VPS4B abundance based on immunoblotting analysis as shown in the upper panel. NT—non-transfected. Data are means of four independent experiments ± SEM. Wilcoxon signed rank test; ns—non-significant (P ≥ 0.05). C. Correlation between dependency scores and VPS4B copy number for selected cancer cell lines from the DepMap portal dataset (https://depmap.org/portal/). According to the portal, a lower score (below −0.5) means that a gene is more likely to be dependent in a given cell line. A score of 0 is equivalent to a gene that is not essential, whereas a score of −1 corresponds to the median of all common essential genes. D. Immunoblotting analysis of VPS4A silencing efficiency in HOP62 and SNU410 cell lines. Lysates were prepared 6 days after siRNA transfection with non-targeting (siCTRL#1) or VPS4A-targeting (siVPS4A#2 or #5) duplexes. Vinculin was used as a loading control. Data information: The exact P-values can be found in Appendix Table S3. Download figure Download PowerPoint Using a short-term viability assay and a long-term colony formation assay, we demonstrated that single depletion of VPS4A or VPS4B did not affect HCT116 growth in vitro (Fig 2A and B). However, simultaneous depletion of VPS4A+B significantly reduced HCT116 viability as indicated by decreased proliferation and colony growth. Moreover, we observed similar effects in other CRC cell lines, such as RKO, SW480, and DLD-1, each harboring various cancer driver mutations. We found impaired cell viability of these lines only when expression of both VPS4 paralogs was silenced simultaneously (Fig 2C). Figure 2. Synthetic lethality between VPS4A and VPS4B inhibits growth of CRC lines in vitro A. Analysis of viability of HCT116 cells assessed 96 h after transfection with independent non-targeting siRNA (two different duplexes used, siCTRL#1 or #2) or targeting VPS4A (duplexes #1 or #2), VPS4B (duplexes #1 or #2), or both VPS4 (various combinations of siVPS4A+siVPS4B duplexes). Data are means of three independent experiments ± SEM. All values were normalized, averaged (avrg) viability of siCTRL#1- and #2-transfected cells was set as 100%, the Kruskal–Wallis test followed by Dunn's multiple comparison post hoc test; ns—non-significant (P ≥ 0.05), **P < 0.01. B. Left panel, analysis of clonogenic growth of HCT116 cells assessed 15 days after transfection with non-targeting (siCTRL#1), VPS4A- or/and VPS4B-targeting siRNAs used in various duplex combinations as indicated. Right panel, images of HCT116 clones taken at the day of clonogenic growth assessment. Data are means of three independent experiments ± SEM. NT—non-transfected. All values were normalized, and clonogenic growth of siCTRL#1-transfected cells was set as 1. One-sample t-test; ns—non-significant (P ≥ 0.05), ****P < 0.0001. C. Viability of RKO and SW480 cells assessed as in (A), growth of DLD-1 cells assessed in BrdU incorporation assay 96 h after siRNA transfection. Various independent non-targeting (siCTRL#1 or #2) and VPS4A- or B-targeting siRNA duplexes were used (#1, #2, #5 or #1, #2, respectively). All values were normalized, and averaged (avrg) viability of siCTRL#1- and #2-transfected cells was set as 100%. Data are means of three independent experiments ± SEM. The Kruskal–Wallis test followed by Dunn's multiple comparison post hoc; ns—non-significant (P ≥ 0.05), **P < 0.01, ***P < 0.001. D. VPS4B copy number and dependency scores of selected cancer cell lines obtained in CRISPR/Cas9 and RNAi screens deposited in the DepMap portal (https://depmap.org/portal/). E. VPS4B copy number status estimated across different cell lines using TaqMan assay. The error bars represent the minimal and maximal copy number in a given triplicate readout. The BCL2 gene localized on 18q in the close vicinity to VPS4B was analyzed as a control. F. VPS4B and VPS4A protein abundance in selected normal and cancer cell lines analyzed by immunoblotting. Vinculin and GAPDH were used as loading controls. G. Analysis of viability of HOP62 lung cancer cells assessed 144 h after transfection with independent non-targeting siRNA (two different duplexes used, siCTRL#1 or #2) or targeting VPS4A (duplexes #2 or #5), VPS4B (duplexes #1 or #2), or both VPS4 (various combinations of siVPS4A+siVPS4B duplexes). Data are means of four independent experiments ± SEM. All values were normalized, and cell viability of averaged (avrg) siCTRL#1- and #2-transfected cells was set as 100%. Two-tailed unpaired t-test; ****P < 0.0001. H. Top panel, analysis of clonogenic growth of HOP62 cells assessed 14 days after transfection with various siRNA duplexes as indicated. Bottom panel, images of HOP62 clones taken at the day of clonogenic growth assessment. Data are means of four independent experiments ± SEM. All values were normalized, and colony area of averaged (avrg) siCTRL#1- and #2-transfected cells was set as 1. The Mann–Whitney U-test; **P < 0.01. I. Analysis of viability of SNU410 pancreatic cancer cells assessed 168 h after transfection with independent non-targeting siRNA (three different duplexes used, siCTRL#1, #2, or #3) or targeting VPS4A (duplexes #2, #4, or #5), VPS4B (duplexes #1 or #2), or both VPS4 (various combinations of siVPS4A+siVPS4B duplexes). Data are means of three independent experiments ± SEM. All values were normalized, cell viability of averaged (avrg) siCTRL#1-, #2-, and #3-transfected cells was set as 100%. Two-tailed unpaired t-test; ****P < 0.0001. Data information: The exact P-values can be found in the source data for this figure. Source data are available online for this figure. Source Data for Figure 2 [emmm201910812-sup-0007-SDataFig2.zip] Download figure Download PowerPoint To confirm the synthetic lethality between VPS4A and VPS4B, we wished to use a non-engineered cancer cell line with low VPS4B expression. To identify such an in vitro model, we took advantage of datasets from the Dependency Map (DepMap) portal (https://depmap.org/portal/),
更多查看译文
关键词
synthetic lethality,colorectal cancer,inflammatory response
AI 理解论文
溯源树
样例
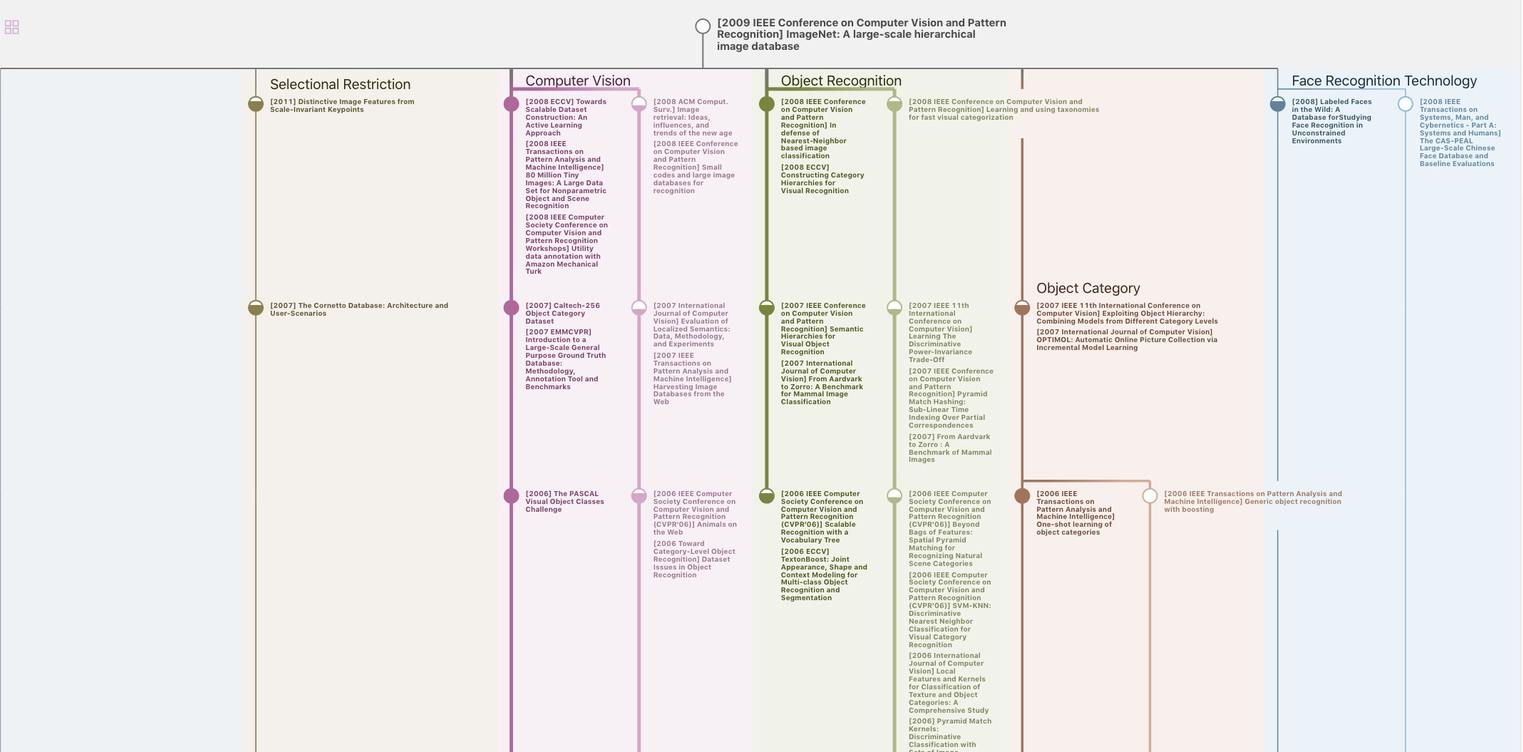
生成溯源树,研究论文发展脉络
Chat Paper
正在生成论文摘要