Modeling of Chemical Membrane Degradation Mitigation and Performance Tradeoffs in Ceria-Supported Fuel Cells
ECS Meeting Abstracts(2016)
摘要
Ceria supported membranes have been proposed to mitigate chemical membrane degradation in polymer electrolyte fuel cells. It was confirmed that ceria as a radical scavenger protects the membrane under open circuit voltage (OCV) or ex-situ Fenton’s durability conditions [1-7]. However, its effectiveness has not been examined for cell voltages below OCV, which are necessary conditions for field operation of fuel cells. On the other hand, ceria membrane additive can be considered as a cation contamination since it is dissolved in the membrane [8]. Performance tradeoffs have been observed experimentally with the use of ceria membrane additive, and the tradeoffs are more significant at lower cell voltages [9]. Therefore, a comprehensive investigation on chemical membrane mitigation and performance tradeoffs in ceria-supported fuel cells is required. In the present work, a transient in-situ chemical degradation model for simulating the transport and reaction of cerium redox couples in the membrane electrode assembly (MEA) is developed and integrated with the chemical membrane degradation models in which the effects of iron redox couples on membrane degradation and detailed ionomer degradation processes are included [10-11].The developed model is then applied to investigate the mitigation effectiveness of cerium redox couples and the fundamental mechanisms for the performance tradeoffs under different cell voltage conditions. The modeling results reveal that abundant Ce(III) ions are available in the membrane to quench hydroxyl radicals at high cell voltages. Since the hydroxyl radical is the dominant reactive species to attack the membrane ionomer, the modeling results demonstrate the primary mechanism for the significant mitigation observed in ceria supported MEAs at OCV conditions. However, this type of mitigation is found to be suppressed at low cell voltages, where electromigration drives Ce(III) ions into the cathode catalyst layer and reduces the available Ce(III) ion in the membrane. Ce(III) ion is the dominant species for hydroxyl radical quenching, and inadequate amount of Ce(III) ion in the membrane leads to a ten-fold reduction in the mitigation effectiveness at cell voltages below 0.7 V. The simulated Ce(III) ion migration to the cathode catalyst layer is found to be responsible for the performance losses observed in ceria supported MEAs. The modeling results reveal that proton starvation can occur in the cathode catalyst layer due to the local Ce(III) ion accumulation. Without the adequate supply of protons in the ionomer of the cathode catalyst layer, proton conductivity and oxygen reduction kinetics are reduced. Significant performance tradeoffs in the form of combined ohmic and kinetic voltage losses are therefore evident and shown to increase with current density. Overall membrane durability and fuel cell performance management is shown to be possible at high cell voltages with the use of ceria membrane additive, where cerium migration and the associated performance loss are insignificant. Unfortunately, at low cell voltages additional steps must be taken to address proton starvation in the cathode catalyst layer and inadequate amount of Ce(III) ion in the membrane in order to achieve a durable membrane without compromising fuel cell performance. Acknowledgement: This research was supported by Ballard Power Systems and the Natural Sciences and Engineering Research Council of Canada through an Automotive Partnership Canada (APC) grant. The authors wish to thank their colleagues at SFU FCReL and Ballard for providing valuable comments and advices. References: [1] Coms et al., ECS Trans. 16 (2) (2008) 1735–1747 [2] Xiao et al., J. Power Sources 195 (16) (2010) 5305 – 5311 [3] Wang et al., J. Membrane Sci. 421422 (0) (2012) 201 – 210 [4] Pearman et al., J. Power Sources 225 (0) (2013) 75 – 83 [5] Pearman et al., Polym. Degard. Stabil. 98 (9) (2013) 1766 – 1772 [6] Wang et al., Electrochimica Acta 109 (0) (2013) 775 – 780 [7] Lim et al., ECS Electrochem. Lett. 4 (4) (2015) F29–F31 [8] Hayes et al., J. Electrochem. Soc. 149(12) (2002) C623–C630 [9] Cheng et al., J. Electrochem. Soc., 160(1) (2013) F27–F33 [10] Wong et al., J. Electrochem. Soc., 161(9) (2014) F823–F832 [11] Wong et al., ChemSusChem, 8(6) (2015) 1072–1082
更多查看译文
AI 理解论文
溯源树
样例
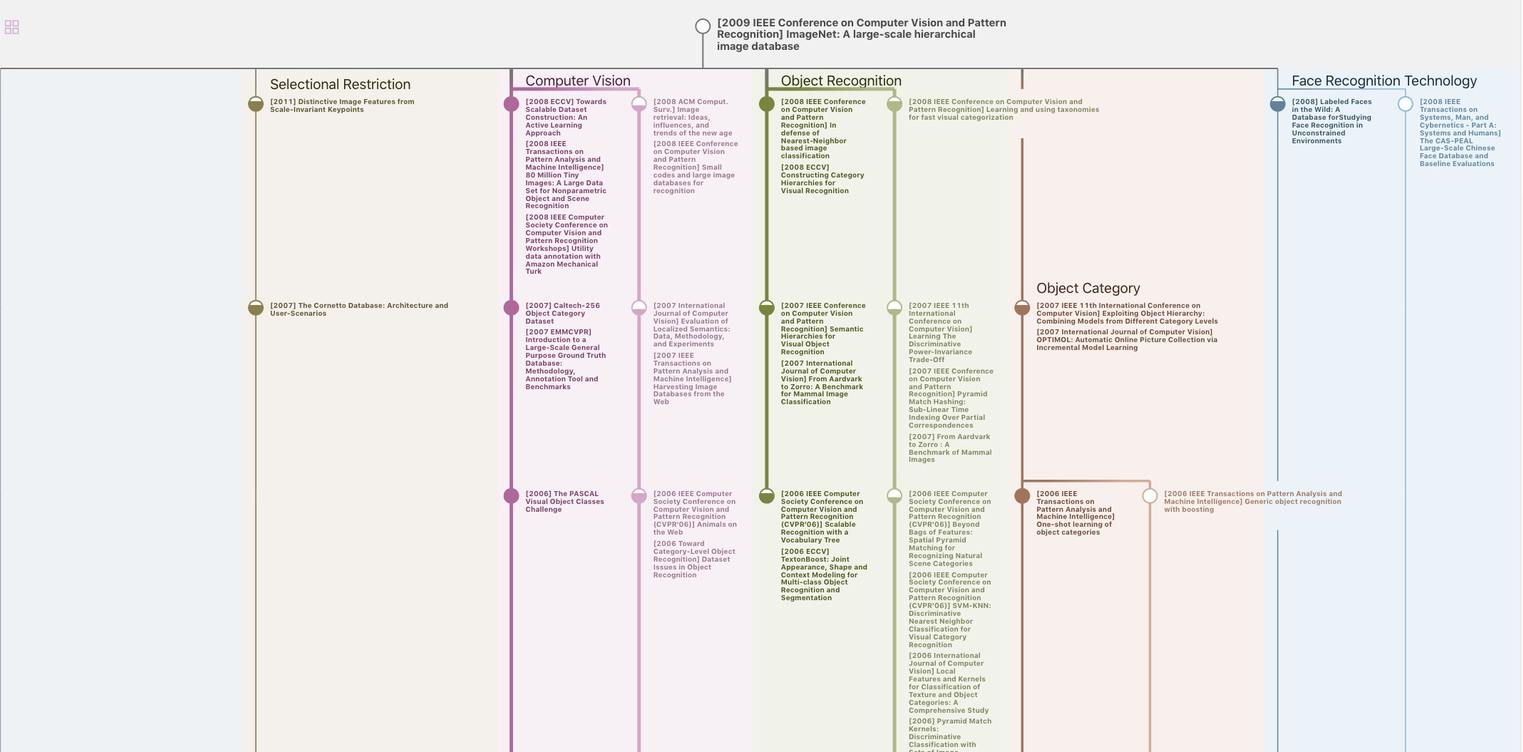
生成溯源树,研究论文发展脉络
Chat Paper
正在生成论文摘要