Hypothalamic
CDK
4 regulates thermogenesis by modulating sympathetic innervation of adipose tissues
EMBO reports(2020)
摘要
Article12 July 2020Open Access Source DataTransparent process Hypothalamic CDK4 regulates thermogenesis by modulating sympathetic innervation of adipose tissues Judit Castillo-Armengol orcid.org/0000-0001-7833-0569 Center for Integrative Genomics, University of Lausanne, Lausanne, Switzerland Search for more papers by this author Valentin Barquissau Center for Integrative Genomics, University of Lausanne, Lausanne, Switzerland Search for more papers by this author Sarah Geller Center for Integrative Genomics, University of Lausanne, Lausanne, Switzerland Search for more papers by this author Honglei Ji Center for Integrative Genomics, University of Lausanne, Lausanne, Switzerland Search for more papers by this author Ilenia Severi Department of Experimental and Clinical Medicine, Marche Polytechnic University, Ancona, Italy Search for more papers by this author Wiebe Venema Department of Experimental and Clinical Medicine, Marche Polytechnic University, Ancona, Italy Search for more papers by this author Eric Aria Fenandez Center for Integrative Genomics, University of Lausanne, Lausanne, Switzerland Search for more papers by this author Catherine Moret Center for Integrative Genomics, University of Lausanne, Lausanne, Switzerland Search for more papers by this author Katharina Huber Center for Integrative Genomics, University of Lausanne, Lausanne, Switzerland Search for more papers by this author Lucia C Leal-Esteban Center for Integrative Genomics, University of Lausanne, Lausanne, Switzerland Search for more papers by this author Anita Nasrallah Center for Integrative Genomics, University of Lausanne, Lausanne, Switzerland Search for more papers by this author Laia Martinez-Carreres Center for Integrative Genomics, University of Lausanne, Lausanne, Switzerland Search for more papers by this author Guy Niederhäuser Center for Integrative Genomics, University of Lausanne, Lausanne, Switzerland Search for more papers by this author Patricia Seoane-Collazo NeurObesity Group, Department of Physiology, CIMUS, University of Santiago de Compostela-Instituto de Investigación Sanitaria, Santiago de Compostela, Spain CIBER Fisiopatología de la Obesidad y Nutrición (CIBEROBN), Santiago de Compostela, Spain Search for more papers by this author Sylviane Lagarrigue Department of Physiology, University of Lausanne, Lausanne, Switzerland Search for more papers by this author Miguel López orcid.org/0000-0002-7823-1648 NeurObesity Group, Department of Physiology, CIMUS, University of Santiago de Compostela-Instituto de Investigación Sanitaria, Santiago de Compostela, Spain CIBER Fisiopatología de la Obesidad y Nutrición (CIBEROBN), Santiago de Compostela, Spain Search for more papers by this author Antonio Giordano Department of Experimental and Clinical Medicine, Marche Polytechnic University, Ancona, Italy Search for more papers by this author Sophie Croizier orcid.org/0000-0002-0076-1008 Center for Integrative Genomics, University of Lausanne, Lausanne, Switzerland Search for more papers by this author Bernard Thorens Center for Integrative Genomics, University of Lausanne, Lausanne, Switzerland Search for more papers by this author Isabel C Lopez-Mejia Center for Integrative Genomics, University of Lausanne, Lausanne, Switzerland Search for more papers by this author Lluis Fajas Corresponding Author [email protected] orcid.org/0000-0002-1283-9503 Center for Integrative Genomics, University of Lausanne, Lausanne, Switzerland Search for more papers by this author Judit Castillo-Armengol orcid.org/0000-0001-7833-0569 Center for Integrative Genomics, University of Lausanne, Lausanne, Switzerland Search for more papers by this author Valentin Barquissau Center for Integrative Genomics, University of Lausanne, Lausanne, Switzerland Search for more papers by this author Sarah Geller Center for Integrative Genomics, University of Lausanne, Lausanne, Switzerland Search for more papers by this author Honglei Ji Center for Integrative Genomics, University of Lausanne, Lausanne, Switzerland Search for more papers by this author Ilenia Severi Department of Experimental and Clinical Medicine, Marche Polytechnic University, Ancona, Italy Search for more papers by this author Wiebe Venema Department of Experimental and Clinical Medicine, Marche Polytechnic University, Ancona, Italy Search for more papers by this author Eric Aria Fenandez Center for Integrative Genomics, University of Lausanne, Lausanne, Switzerland Search for more papers by this author Catherine Moret Center for Integrative Genomics, University of Lausanne, Lausanne, Switzerland Search for more papers by this author Katharina Huber Center for Integrative Genomics, University of Lausanne, Lausanne, Switzerland Search for more papers by this author Lucia C Leal-Esteban Center for Integrative Genomics, University of Lausanne, Lausanne, Switzerland Search for more papers by this author Anita Nasrallah Center for Integrative Genomics, University of Lausanne, Lausanne, Switzerland Search for more papers by this author Laia Martinez-Carreres Center for Integrative Genomics, University of Lausanne, Lausanne, Switzerland Search for more papers by this author Guy Niederhäuser Center for Integrative Genomics, University of Lausanne, Lausanne, Switzerland Search for more papers by this author Patricia Seoane-Collazo NeurObesity Group, Department of Physiology, CIMUS, University of Santiago de Compostela-Instituto de Investigación Sanitaria, Santiago de Compostela, Spain CIBER Fisiopatología de la Obesidad y Nutrición (CIBEROBN), Santiago de Compostela, Spain Search for more papers by this author Sylviane Lagarrigue Department of Physiology, University of Lausanne, Lausanne, Switzerland Search for more papers by this author Miguel López orcid.org/0000-0002-7823-1648 NeurObesity Group, Department of Physiology, CIMUS, University of Santiago de Compostela-Instituto de Investigación Sanitaria, Santiago de Compostela, Spain CIBER Fisiopatología de la Obesidad y Nutrición (CIBEROBN), Santiago de Compostela, Spain Search for more papers by this author Antonio Giordano Department of Experimental and Clinical Medicine, Marche Polytechnic University, Ancona, Italy Search for more papers by this author Sophie Croizier orcid.org/0000-0002-0076-1008 Center for Integrative Genomics, University of Lausanne, Lausanne, Switzerland Search for more papers by this author Bernard Thorens Center for Integrative Genomics, University of Lausanne, Lausanne, Switzerland Search for more papers by this author Isabel C Lopez-Mejia Center for Integrative Genomics, University of Lausanne, Lausanne, Switzerland Search for more papers by this author Lluis Fajas Corresponding Author [email protected] orcid.org/0000-0002-1283-9503 Center for Integrative Genomics, University of Lausanne, Lausanne, Switzerland Search for more papers by this author Author Information Judit Castillo-Armengol1, Valentin Barquissau1, Sarah Geller1, Honglei Ji1, Ilenia Severi2, Wiebe Venema2, Eric Aria Fenandez1, Catherine Moret1, Katharina Huber1, Lucia C Leal-Esteban1, Anita Nasrallah1, Laia Martinez-Carreres1, Guy Niederhäuser1, Patricia Seoane-Collazo3,4, Sylviane Lagarrigue5, Miguel López3,4, Antonio Giordano2, Sophie Croizier1, Bernard Thorens1, Isabel C Lopez-Mejia1 and Lluis Fajas *,1 1Center for Integrative Genomics, University of Lausanne, Lausanne, Switzerland 2Department of Experimental and Clinical Medicine, Marche Polytechnic University, Ancona, Italy 3NeurObesity Group, Department of Physiology, CIMUS, University of Santiago de Compostela-Instituto de Investigación Sanitaria, Santiago de Compostela, Spain 4CIBER Fisiopatología de la Obesidad y Nutrición (CIBEROBN), Santiago de Compostela, Spain 5Department of Physiology, University of Lausanne, Lausanne, Switzerland *Corresponding author. Tel: +41 21 692 41 11; E-mail: [email protected] EMBO Rep (2020)21:e49807https://doi.org/10.15252/embr.201949807 PDFDownload PDF of article text and main figures. Peer ReviewDownload a summary of the editorial decision process including editorial decision letters, reviewer comments and author responses to feedback. ToolsAdd to favoritesDownload CitationsTrack CitationsPermissions ShareFacebookTwitterLinked InMendeleyWechatReddit Figures & Info Abstract This study investigated the role of CDK4 in the oxidative metabolism of brown adipose tissue (BAT). BAT from Cdk4−/− mice exhibited fewer lipids and increased mitochondrial volume and expression of canonical thermogenic genes, rendering these mice more resistant to cold exposure. Interestingly, these effects were not BAT cell-autonomous but rather driven by increased sympathetic innervation. In particular, the ventromedial hypothalamus (VMH) is known to modulate BAT activation via the sympathetic nervous system. We thus examined the effects of VMH neuron-specific Cdk4 deletion. These mice display increased sympathetic innervation and enhanced cold tolerance, similar to Cdk4−/− mice, in addition to browning of scWAT. Overall, we provide evidence showing that CDK4 modulates thermogenesis by regulating sympathetic innervation of adipose tissue depots through hypothalamic nuclei, including the VMH. This demonstrates that CDK4 not only negatively regulates oxidative pathways, but also modulates the central regulation of metabolism through its action in the brain. Synopsis CDK4 negatively regulates thermogenesis by suppressing oxidative pathways. This study reveals that CDK4 also modulates the systemic regulation of metabolism through its action in the hypothalamus. CDK4−/− mice exhibit increased energy expenditure and are cold resistant. BAT specific CDK4 deletion does not affect thermogenesis. CDK4−/− mice display increased sympathetic innervation of BAT and browning of subcutaneous WAT. VMH SF1 neuron specific CDK4−/− mice show increased adrenergic signaling and brown adipose tissue innervation. CDK4−/− mice exhibit increased sympathetic activity in the ventromedial hypothalamus. Introduction Growing evidence demonstrates that a regulatory crosstalk exists between metabolic pathways and regulators of cell cycle progression. Our laboratory and others have demonstrated that CDK4 is one of such “metabolic” cell cycle regulators (Blanchet et al, 2011; Denechaud et al, 2016; Lagarrigue et al, 2016; Lopez-Mejia et al, 2017). CDK4 participates in the G1/S transition phase of the cell cycle. Growth factors or mitotic stimuli activate this kinase by promoting its interaction with D-type cyclins, ultimately resulting in the activation of the E2F transcription factors, which regulate the expression of genes important for DNA synthesis and chromosome duplication (Malumbres, 2014). In addition to, or in complement to, cell cycle regulation, CDK4 represses the oxidative metabolism indirectly, via the control of the E2F1 transcription factor in muscle and brown adipose tissue (BAT) (Blanchet et al, 2011), and promotes the insulin signaling pathway in mature adipocytes (Lagarrigue et al, 2016). Interestingly, we previously showed that the AMP-activated protein kinase (AMPK), which is a central inhibitor of such anabolic processes, is a direct target of, and is repressed by, CDK4 (Lopez-Mejia et al, 2017). Overall, the participation of cell cycle regulators in the control of energy homeostasis occurs mainly through the activation of anabolic processes and the repression of the oxidative metabolism. Because of the role of CDK4 in the regulation of mitochondrial activity and in the overall oxidative metabolism in MEFs (Lopez-Mejia et al, 2017), we investigated the function of CDK4 in BAT biology, which is a tissue in which oxidative metabolism is central for its thermogenic function. Adipose tissue has a major contribution to the regulation of energy homeostasis. Distinct adipose tissue types have different functions in the overall regulation of metabolism. Essentially, three classes of adipose tissue can be distinguished: white adipose tissue (WAT), which stores energy in the form of triacylglycerols (TAG), BAT that dissipates energy in the form of heat, and beige adipose tissue that also generates heat, but in contrast to BAT is embedded into WAT, and has a specific gene expression pattern (Giralt & Villarroya, 2013). Brown adipose tissue is unique in that its activation can increase energy expenditure and glucose clearance (Lowell et al, 1993; Harper et al, 2001; Cannon & Nedergaard, 2009). BAT is a highly specialized thermogenic organ which acts to maintain body temperature during cold stress (Cannon & Nedergaard, 2004) and is composed of brown adipocytes that contain numerous lipid droplets and a high number of mitochondria which express uncoupling protein 1 (UCP1). UCP1 uncouples ATP production from mitochondrial respiration to produce heat (Cannon & Nedergaard, 2004; Fedorenko et al, 2012). These characteristics suggest that the modulation of BAT activity may be harnessed therapeutically to combat obesity and diabetes. Although initially described in small mammals in the 16th century, evidence of metabolically active BAT depots in adult humans only came to light more recently (Nedergaard et al, 2007). Cold perception is mediated by sensory neurons found in several parts of the body, such as the skin, which in turn relays their information to the central nervous system (CNS) (Morrison et al, 2012, 2014). There, several hypothalamic nuclei integrate this sensory information to regulate the thermogenic response (Oldfield et al, 2002; Monda et al, 2005; Morrison et al, 2012; Contreras et al, 2015; Martins et al, 2016; Lee et al, 2018). Among them, the ventromedial nucleus of the hypothalamus (VMH) was one of the first nuclei described as a regulator of thermogenesis (Perkins et al, 1981). Indeed, a major population of VMH neurons which express the transcription factor steroidogenic factor-1 (SF1) has been shown to contribute to BAT activation, and thus thermogenesis, by modulating its sympathetic stimulation (Kim et al, 2011; Contreras et al, 2015; Labbe et al, 2015; Martinez-Sanchez et al, 2017; Seoane-Collazo et al, 2018). The sympathetic nervous system directly innervates BAT to control heat production (De Matteis et al, 1998; Murano et al, 2009; Bartness et al, 2010; Lopez et al, 2010). Tyrosine hydroxylase-expressing nervous fibers (TH fibers) in BAT secrete norepinephrine, the principal stimulator of BAT activity, locally (Bartness et al, 2010). Norepinephrine in turn stimulates β-adrenergic receptors in brown adipocytes to increase intracellular cAMP levels via the adenylate cyclase and activate the PKA pathway, thus increasing lipid mobilization and thermogenesis (Collins & Surwit, 2001). Moreover, sympathetic innervation also increases mitochondrial number and activity (Klingenspor et al, 1996; Puigserver et al, 1998; Sanchez-Alavez et al, 2013). In the present study, we show that Cdk4 knockout mice (Cdk4nc/nc, referred to in this manuscript as Cdk4−/−) (Martin et al, 2003; Lagarrigue et al, 2016) are resistant to cold. By using two additional mouse models, in which we deleted Cdk4 specifically in BAT or in the VMH SF1 neurons, we prove that these effects are mediated by the action of CDK4 in the hypothalamus, and raise the possibility of a broader function for CDK4 in other brain areas. Results Cdk4 knockout mice show increased energy expenditure, improved adaptation to cold, and increased mitochondrial volume Cdk4 knockout mice (Cdk4−/−) were first used to explore the role of CDK4 in BAT metabolism. As previously described, Cdk4−/− mice were smaller (Fig EV1A) and had decreased body mass and decreased fat mass compared to Cdk4+/+ mice (Fig EV1B and C) (Lagarrigue et al, 2016). Despite their smaller size and leaner phenotype, these mice showed a 20% increase in food intake compared to Cdk4+/+ mice when corrected by body weight (Fig EV1D and E). Moreover, relative to control mice, at room temperature (24°C), Cdk4−/− mice showed a significant increase in oxygen consumption (Fig 1A and B) and energy expenditure (EE; Fig 1C and D). The respiratory exchange ratio (RER) was slightly decreased, namely during the dark phase (Fig 1E and F), in Cdk4−/− compared to Cdk4+/+ mice, suggesting that these mice preferentially used fatty acids as energy substrates. Click here to expand this figure. Figure EV1. Cdk4−/− mice are smaller and have increased food intake compared to their wild-type littermates (Cdk4+/+) A. Whole-body image of Cdk4+/+ and Cdk4−/− mice. B, C. Body weight (B) and fat mass percentage (C) of Cdk4+/+ (n = 5) and Cdk4−/− (n = 6) mice. D, E. Food intake was measured using the Phenomaster device (TSE system) and is shown as cumulative intake over 24 h (D) and daily cumulative food intake (E) of 14-week-old Cdk4+/+ (n = 7) and Cdk4−/− (n = 8) mice. Data information: All data are shown as the mean ± SEM. Student's t-test was used for statistical analysis *P < 0.05; ***P < 0.001. Source data are available online for this figure. Download figure Download PowerPoint Figure 1. Increased whole-body oxygen consumption and lipid utilization, and improved resistance to cold exposure in Cdk4−/− mice A–F. Indirect calorimetry was performed using the Oxymax/CLAMS system in Cdk4+/+ (n = 6) and Cdk4−/− (n = 5) mice. Whole-body oxygen consumption rate (VO2) (A, B), energy expenditure (EE) (C, D), and respiratory exchange ratio (RER) (E, F) were measured at 24°C and during a cold challenge at 6°C during both the light (white rectangle) and dark (black rectangle) phases over a 2-day period. G–I. CalR was used to implement GLM-regression plot for each group corresponding to the association between energy balance and total body mass at 24°C (G) and the association between energy balance and total body mass at 6°C (H). The CalR interface displays each mouse as a dot, and the standard error of mean for each group in gray. The “mass effect” and “group effect” were analyzed using a generalized linear model (GLM) using body weight as a covariate. The results of this analysis are shown in table (I) for Cdk4+/+ (n = 6) and Cdk4−/− (n = 5) mice. J, K. Basal rectal temperature was measured at 24°C (J), and rectal temperature was monitored during acute cold exposure at 4°C for 6 h (K) in Cdk4+/+ (n = 10) and Cdk4−/− (n = 8) mice. Data information: All data are shown as the mean ± SEM; Student's t-test was used for statistical analysis. *P < 0.05, **P < 0.01, ***P < 0.001. Source data are available online for this figure. Source Data for Figure 1 [embr201949807-sup-0003-SDataFig1.xlsx] Download figure Download PowerPoint Considering that the activation of BAT is known to increase whole-body energy expenditure by increasing thermogenesis (Cannon & Nedergaard, 2009), we hypothesized that the increased EE we observed in the Cdk4−/− mice could be explained by increased thermogenesis. Indeed, BAT activity is specifically increased during cold exposure in order to maintain body temperature. Thus, to explore the activation of BAT in Cdk4−/− mice, we exposed the mice to a 6°C challenge and measured oxygen consumption, EE, and the RER by indirect calorimetry. Cold exposure (from 24 to 6°C) increased oxygen consumption (Fig 1A and B) and EE (Fig 1C and D), and decreased RER (Fig 1E and F) in the control Cdk4+/+ animals. Compared to controls, at 6°C Cdk4−/− mice showed higher levels of oxygen consumption (Fig 1A and B), increased EE (Fig 1C and D), and a greater decrease in RER (Fig 1E and F), indicating increased lipid utilization. In addition, when the energy balance was calculated, Cdk4−/− mice clearly displayed a negative energy balance, at room temperature and upon cold exposure (Fig 1G and H), which is significantly different from the behavior observed in Cdk4+/+ mice (Fig 1I). These data suggested that Cdk4−/− mice are primed to better respond to energy stress situations, like cold exposure than their control littermates. To test this hypothesis, we next exposed the animals to acute cold (4°C) and followed their body temperature for 6 h. The basal rectal temperature of Cdk4−/− mice was similar to that of their control littermates (Fig 1J). However, Cdk4−/− mice maintained a consistently higher body temperature than controls during the 6 h of cold exposure (Fig 1K). These results suggest that the depletion of CDK4 results in increased thermogenesis in response to cold. To understand how CDK4 depletion affects thermogenesis, we next examined the effect of global Cdk4 deletion on the morphology and thermogenic activity of interscapular BAT (iBAT). In Cdk4−/− mice, the iBAT mass was decreased and the tissue was visibly darker than in controls (Fig 2A and B), characteristics suggestive of higher oxidative activity. Indeed, morphological analysis of hematoxylin–eosin-stained iBAT revealed a decrease in lipid droplet size in Cdk4−/− animals compared to droplet size in Cdk4+/+ mice (Fig 2C). However, analysis of the expression levels of the phosphorylated lipase protein (p-HSL S660) in BAT protein lysates showed no differences, suggesting a compensatory effect in HSL activity due to overactivation (Appendix Fig S3C and D). Figure 2. Cdk4 deficiency promotes thermogenesis and mitochondrial biogenesis in iBAT A. Gross morphology of iBAT from Cdk4+/+ and Cdk4−/− mice. B. iBAT mass normalized to body weight of Cdk4+/+ (n = 11) and Cdk4−/− (n = 10) mice. C. Hematoxylin–eosin staining and UCP1 immunohistochemical (IHC) staining of iBAT sections (scale bar 100 μm) from Cdk4+/+ and Cdk4−/− mice. D, E. Western blot analysis of UCP1 and CDK4 protein expression (D) and quantified protein levels of UCP1 (E) (n = 4 biological replicates) in iBAT. HSP90 was used as loading control. F. Expression of thermogenic genes and brown adipocyte markers in iBAT of Cdk4+/+ (n = 6) and Cdk4−/− (n = 6) mice as assessed by RT–PCR. G, H. Transmission electron microscopy (TEM) images of iBAT (G) (scale bar 5 μm [upper panel] and 1 μm [lower panel, LD indicates lipid droplet and M mitochondria]) and quantification of lipid droplet number (LD) and mitochondrial volume (Mito) (H) (Cdk4+/+ [n = 4] and Cdk4−/− [n = 4]). I. Respirometry analysis of iBAT as measured by the Oroboros system (Cdk4+/+ [n = 6] and Cdk4−/− [n = 6]). Data information: All data are shown as the mean ± SEM; Student's t-test (B, F, H, I) and Mann–Whitney U-test (E) were used for statistical analyses. *P < 0.05, **P < 0.01. Source data are available online for this figure. Source Data for Figure 2 [embr201949807-sup-0004-SDataFig2.zip] Download figure Download PowerPoint We also observed increased expression of UCP1 in the iBAT of Cdk4−/− mice by immunohistochemistry (Fig 2C) and western blot (Fig 2D and E), further suggesting that these animals displayed increased oxidative activity and thermogenesis. Moreover, mRNA level analysis by RT–qPCR revealed a significant upregulation of thermogenic genes and brown adipocyte markers in the Cdk4−/− mice relative to controls, with Ucp1, Dio2, and Bmp8b being especially highly increased (Fig 2F). Taken together, these results suggested that Cdk4-deficient mice display a marked prothermogenic phenotype. To further investigate potential subcellular metabolic changes induced by Cdk4 knockout, we next examined iBAT mitochondrial morphology and function. We observed a significant 40% increase in mitochondrial volume and visibly decreased lipid content in the iBAT of Cdk4−/− mice, as measured by transmission electron microscopy (TEM; Fig 2G and H). Next, we determined the oxidative capacity of iBAT by respirometry analysis using the Oroboros system. Coupled respiration through complexes I and II (CI + CII) and maximal electron transport system capacity were significantly increased in iBAT homogenates from the Cdk4−/− mice relative to controls (Fig 2I). Taken together, these results suggest that Cdk4−/− mice have a higher thermogenic capacity due to an increased mitochondrial volume and function, which in turn increases the ability of BAT to uncouple mitochondria and generate heat. BAT-specific deletion of Cdk4 has no impact on thermogenesis To determine whether the deletion of Cdk4 from BAT in particular was responsible for the increased thermogenesis observed in Cdk4−/− mice, and to further explore the role of CDK4 in BAT, we generated BAT-specific Cdk4 knockout mice by crossing Cdk4flox/flox mice with Ucp1-Cre transgenic mice, which express the Cre recombinase specifically in brown adipocytes, thus generating Cdk4flox/flox Ucp1-Cre mice. Cdk4flox/flox Ucp1-Cre animals, compared to controls, had lower CDK4 levels in iBAT, but normal CDK4 levels in subcutaneous WAT (scWAT) and perigonadal WAT (pgWAT), demonstrating the tissue selectivity of the knockout (Fig EV2A). However, no differences in body size (Fig EV2B), body weight (Fig EV2C), or body composition (Fig EV2D) were observed in Cdk4flox/flox Ucp1-Cre mice compared to those of their control littermates. Moreover, no differences were observed in body temperature at 24°C (Fig 3A) or during cold exposure (4°C for 6 h; Fig 3B) between Cdk4flox/flox Ucp1-Cre and Cdk4flox/flox mice. In contrast to the Cdk4−/− mice, the iBAT mass, and color from Cdk4flox/flox Ucp1-Cre mice, was similar to that of the controls (Fig 3C and D), with no visible differences in the lipid content, UCP1 staining, or the overall morphology of this tissue between the two genotypes (Fig 3E). Likewise, western blot analysis of UCP1 (Fig 3F and G) and RT–qPCR analysis of thermogenic and BAT-specific gene expression levels (Fig 3H) showed no significant difference in protein or gene expression between the two genotypes. These results demonstrated that CDK4 signaling in BAT does not regulate the thermogenic function of brown adipocytes. Click here to expand this figure. Figure EV2. Cdk4 BAT-specific knockout mice have normal body weight, fat mass, and lean mass A. Western blot for CDK4 expression in iBAT, scWAT, and pgWAT from Cdk4flox/flox (n = 3 biological replicates) and Cdk4flox/flox Ucp1-Cre (n = 3) mice. Tubulin was used as loading control. Please notice that upon amplification, the western blot images are abnormally pixelated. This is because the saturation of the images is shown in FigEV2A_Exposure_3.0sec.scn, which is a direct acquisition file from the Imager, and is found in the data source folder. A JPEG image is also available in this folder. B. Whole-body image of Cdk4flox/flox and Cdk4flox/flox Ucp1-Cre mice. C, D. Body weight (C) and body composition (fat and lean mass) (D) of Cdk4flox/flox (n = 8) and Cdk4flox/flox Ucp1-Cre (n = 8) mice. Data information: All data are shown as the mean ± SEM. Source data are available online for this figure. Download figure Download PowerPoint Figure 3. BAT-specific Cdk4 deletion does not affect the thermogenic characteristics of iBAT A, B. Rectal temperature was measured at 24°C (A), and rectal temperature was monitored for 6 h during acute cold exposure at 4°C (B) in Cdk4flox/flox (n = 9) and Cdk4flox/flox Ucp1-Cre mice (n = 9). C. Gross morphology of iBAT from Cdk4flox/flox and Cdk4flox/flox Ucp1-Cre mice D. iBAT mass compared to body weight of Cdk4flox/flox (n = 8) and Cdk4flox/flox Ucp1-Cre (n = 7) mice. E. Hematoxylin–eosin (H&E) staining and UCP1 immunohistochemical (IHC) staining of iBAT sections (scale bar 100 μm [upper panel] and 50 μm [lower panel]) from Cdk4flox/flox and Cdk4flox/flox Ucp1-Cre mice. F, G. Western blot analysis of UCP1 and CDK4 protein expression (F) and quantified protein levels of UCP1 (G) (n = 4 biological replicates) in iBAT. HSP90 was used as a loading control. H. Expression of thermogenic genes and brown adipocyte markers in iBAT of Cdk4flox/flox (n = 5) and Cdk4flox/flox Ucp1-Cre (n = 6) mice as assessed by RT–PCR. Data information: All data are shown as the mean ± SEM. Student's t-test was used for statistical analysis. ***P < 0.001. Source data are available online for this figure. Source Data for Figure 3 [embr201949807-sup-0005-SDataFig3.zip] Download figure Download PowerPoint Cdk4−/− mice show increased sympathetic innervation of BAT and increased browning of subcutaneous WAT Since CDK4 signaling does not directly regulate thermogenic function in BAT, we next focused on exploring alternative mechanisms. It has been well established that BAT-mediated thermogenesis is regulated by sympathetic innervation (Bartness et al, 2010). TH-positive sympathetic fibers are found in BAT and release norepinephrine, which is required for BAT activation. We hypothesized that an increase in sympathetic innervation may underlie the increase in EE and thermogenesis observed in the Cdk4−/− mice. Indeed, Cdk4-deficient mice had a significantly higher number of TH-positive fibers in iBAT than wild-type mice, as shown by immunohistochemical analysis (Fig 4A and B). The apparent decrease of TH protein levels found when analyzed its expression by western blotting (Appendix Fig S3A and B) is explained by the unbalance in protein concentration which is notably higher in the BAT of Cdk4−/− mice (Appendix Fig S3E). Overcoming this issue by analyzing equivalent amounts of tissue for both genotypes, we determined that TH protein levels were higher in the BAT of Cdk4−/− mice. Similarly, both the synaptic protein PSD95 and UCP1 were also increased in these conditions (Appendix Fig S3F and I). Figure 4. Activation of thermogenesis by increased sympathetic innervation of BAT in Cdk4−/− mice is blunted by β-adrenergic antagonist treatment A, B. TH immunohistochemical staining in iBAT sections (A) (scale bar 20 μm, arrows indicate TH parenchymal fibers) and cor
更多查看译文
关键词
adipose tissues,sympathetic innervation,thermogenesis
AI 理解论文
溯源树
样例
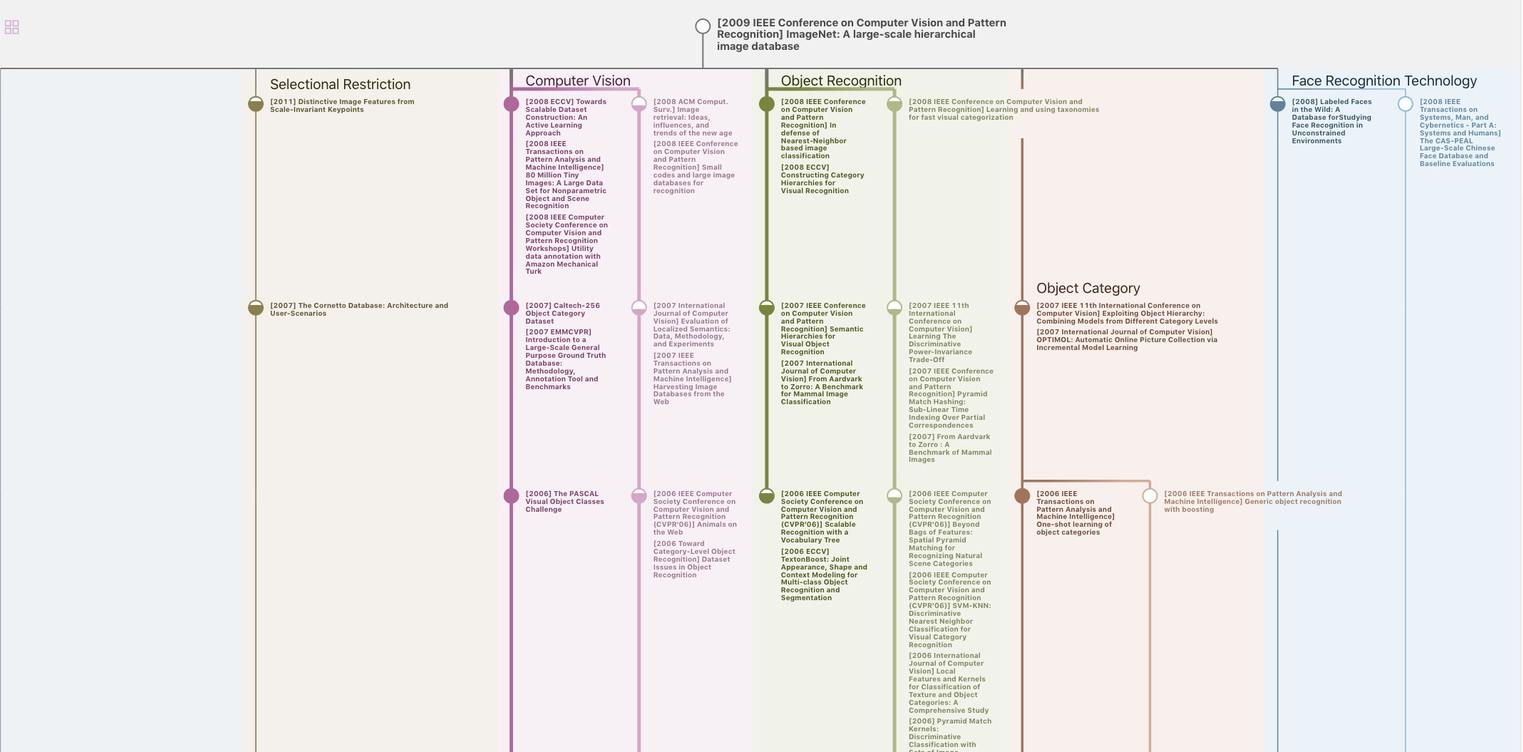
生成溯源树,研究论文发展脉络
Chat Paper
正在生成论文摘要