Particulates are everywhere, but are they harmful in cell and gene therapies?
Cytotherapy(2022)
摘要
Cell and gene therapies (CGTs) are a broad class of revolutionary new treatment modalities with enormous potential to impact multiple intractable diseases. In recent years, the sector has reached a breakthrough point, with unprecedented approvals of cell- and gene-based treatments such as adipose-derived mesenchymal stromal cells (Alofisel, manufactured by Takeda Pharma A/S), chimeric antigen receptor T-cell therapies (e.g., Yescarta, Kite Pharma) and gene therapies (e.g., Luxturna, Spark Therapeutics) and lipid nanoparticle (LNP) vaccines against severe acute respiratory syndrome coronavirus 2 virus (Spikevax by Moderna and Comirnaty by Pfizer-BioNTech). However, when considering particle separation and product purification strategies, these diverse and complex product types pose new manufacturing challenges compared with their biologic (monoclonal antibodies and recombinant proteins) and small-molecule predecessors. These include characterization of particulates generated in the manufacturing process, understanding how these particles impact the safety and efficacy of the therapy and what standards regulators expect various CGT manufacturers to meet. Particulates in therapeutic preparations can vary widely in terms of size and are composed of undissolved organic or inorganic materials that are intrinsic to the drug itself, inherent to the manufacturing process or extrinsically derived from a contaminating source (Table 1) [[1]Langille SE Particulate matter in injectable drug products.PDA J Pharm Sci Technol. 2013; 67: 186-200Crossref PubMed Scopus (68) Google Scholar]. Although large particulates can be removed via filtration or centrifugation methods under current regulatory guidance, smaller subvisible particles measuring 25 μm or less are a concern because of their potential to cause patient harm [[2]Shekunov BY Chattopadhyay P Tong HHY Chow AHL. Particle Size Analysis in Pharmaceutics: Principles, Methods and Applications.Pharmaceutical Research. 2006; 24: 203-227Crossref PubMed Scopus (355) Google Scholar] and the difficulty associated with separating them from the drug product. Additional challenges are presented when the drug product itself can be considered a particulate, as is the case for many advanced therapeutics, such as living cells [[3]Jere D Sediq AS Huwyler J Vollrath I Kardorff M Mahler HC Challenges for Cell-Based Medicinal Products From a Pharmaceutical Product Perspective.J Pharm Sci. 2021; 110: 1900-1908Abstract Full Text Full Text PDF PubMed Scopus (8) Google Scholar], viral particles [[4]Bulaklak K Gersbach CA. The once and future gene therapy.Nature Communications. 2020; 11: 1-4Crossref PubMed Scopus (67) Google Scholar], drug components encapsulated in lipid vesicles [[5]Hou X Zaks T Langer R Dong Y. Lipid nanoparticles for mRNA delivery.Nature Reviews Materials. 2021; 2021: 1-17Google Scholar] and extracellular vesicles (EVs) released from mesenchymal stromal cells [[6]Phinney DG Pittenger MF Concise Review: MSC-Derived Exosomes for Cell-Free Therapy.Stem Cells. 2017; 35: 851-858Crossref PubMed Scopus (882) Google Scholar] or in combination (device–drug) products [[7]Vargason AM Anselmo AC Mitragotri S. The evolution of commercial drug delivery technologies.Nature Biomedical Engineering. 2021; 5: 951-967Crossref PubMed Scopus (157) Google Scholar].Table 1Typical particles found in parenteral drug products.TypeSizeSourceInherentProtein aggregatesSubvisibleDrug formulationAir bubblesVisibleDrug formulationCell aggregatesVisibleDrug productCell debrisSubvisibleDrug substanceIntrinsicBeadsSubvisibleManufacturing processesMicro-carriersVisibleManufacturing processesPolymericVisibleSingle-use plastics and closuresElastomericVisibleContainers and transfer devicesExtrinsicPPE-derivedVisibleGowns, gloves and other PPEDustVisibleRoom airSilicone oilSubvisibleSyringe componentsPolymer fibersVisibleFiltrationRaw materialsSubvisible and visibleContainers and transfer tubingMetalVisibleManufacturing equipmentSuspended particlesSubvisible and visibleRaw materialsPPE, personal protective equipment. Open table in a new tab PPE, personal protective equipment. A typical approach when developing products intended for human use (e.g., medical devices) is to implement clean manufacturing processes to minimize particulate generation and contamination. This approach allows for careful consideration to add cleaning or filtration processes as mitigations where required. Because of the lack of applicable standards, best practices currently involve implementation of process controls to limit and characterize particulates within the product system followed by assessment of final (or clinically representative) product biocompatibility in relevant in vitro tests and/or in vivo models to verify product safety. Of top concern are the addressable risks particles pose to patients [[8]Bukofzer S Ayres J Chavez A Devera M Miller J Ross D et al.Industry Perspective on the Medical Risk of Visible Particles in Injectable Drug Products.PDA Journal of Pharmaceutical Science and Technology. 2015; 69: 123-139Crossref PubMed Scopus (41) Google Scholar]. Particle size is important because drug products delivered directly into the circulation may contain smaller particles measuring 6–8 μm that then become lodged in capillaries [[9]Lehr HA Brunner J Rangoonwala R Kirkpatrick CJ Particulate matter contamination of intravenous antibiotics aggravates loss of functional capillary density in postischemic striated muscle.Am J Respir Crit Care Med. 2002; 165: 514-520Crossref PubMed Scopus (80) Google Scholar]. Similarly, one could envisage that inorganic particulate matter may accumulate in phagocytic cells, which eventually perish, releasing sequestered particulate matter back into the tissue environment and/or potentially causing inflammatory reactions, as demonstrated with nanoparticles [[10]Gustafson HH Holt-Casper D Grainger DW Ghandehari H Nanoparticle Uptake: The Phagocyte Problem.Nano Today. 2015; 10: 487-510Crossref PubMed Scopus (713) Google Scholar]. In addition, repeated intravenous injections or local administrations at the same anatomical region may lead to accumulated particles within local tissues, contributing to chronic inflammation or other deleterious responses [[11]Perez M Maiguy-Foinard A Barthélémy C Décaudin B Odou P. Particulate Matter in Injectable Drugs: Evaluation of Risks to Patients.Pharmaceutical Technology in Hospital Pharmacy. 2016; 1: 91-103Crossref Google Scholar,[12]Jack T Brent BE Boehne M Mü B Sewald K Braun A Analysis of particulate contaminations of infusion solutions in a pediatric intensive care unit.Intensive Care Med. 2010; 36: 707-711Crossref PubMed Scopus (39) Google Scholar]. For all the potential harms presented by particulates, an obvious mitigation is to limit particle generation by focusing on reducing particle size and quantity to the lowest reasonable levels depending on the nature of the drug product, manufacturing process and packaging. Practical guidance on navigating particulate risks in advanced therapy medicinal products would necessitate the measurement of particles generated along the single-use value chain in a typical drug product specific use case. The collection of case studies by drug product and method of particulate monitoring used in the typical manufacturing process would benefit therapy developers, suppliers and service providers. In addition, more comprehensive analysis of particulate-related side effects could be performed by the health care community. Table 2 illustrates some potential adverse effects to consider when assessing particulate risks along with additional mitigation strategies.Table 2Potential harms and mitigations related to particulates in human drug products.Potential harmMitigationTissue implantation Local inflammationCharacterize particulates (inert or biodegradable, biocompatible materials may be acceptable) Local tissue toxicity (necrosis) Pyrogenicity Allergic response (e.g., anaphylaxis due to latex particulates from PPE)Tissue-specific, including immune-privileged sites (brain, spinal cord and eyes) Eye (particulates injected into vitreous humor cause visible “floaters”)Limit visible and opaque or colored particlesBrain and spinal cord Occlusion of arachnoid villi restricts absorption of cerebrospinal fluid Chronic inflammation due to reduced immune response (e.g., limited particle phagocytosis)Limit particulate size and quantityAccess to or communication with vascular system Embolism (particulates clog blood vessels) Thrombus formation (particulates cause clot formation) Thromboembolism (particulates cause clot that embolizes)A cell product itself may present a higher embolic risk; in vivo safety testing should address this Hemocompatibility testingPPE, personal protective equipment. Open table in a new tab PPE, personal protective equipment. Current regulatory guidance provides recommendations on how to reduce particulate load through chemistry, manufacturing and control process improvements, but these standards have largely been written for and applied to traditional pharmaceuticals and biologics. Clarke et al. [[13]Clarke D Stanton J Powers D Karnieli O Nahum S Abraham E et al.Managing particulates in cell therapy: Guidance for best practice.Cytotherapy. 2016; 18: 1063-1076Abstract Full Text Full Text PDF PubMed Scopus (12) Google Scholar] outlined the risk mitigation strategies that could be considered to reduce particulate load in CGT products. However, in the last 5 years, the field has expanded dramatically, with unprecedented approvals. There is now a greater need to move the discussion toward particulate management in cell- and gene-specific regulatory guidance. As CGTs move through the clinical pipeline toward approval and commercial-scale manufacturing, the risk of product contamination with particulate matter needs to be carefully considered. The following key factors, which set advanced therapies apart with respect to particulate identification and removal, need to be considered. Visible and subvisible particles are defined as particles measuring ≥100 μm and <100 μm, respectively (Table 1) [[1]Langille SE Particulate matter in injectable drug products.PDA J Pharm Sci Technol. 2013; 67: 186-200Crossref PubMed Scopus (68) Google Scholar]. Cell types typically used in cell-based therapies, including both hematopoietic and stromal variants, can range from 5 μm to 15 μm, with some larger cells, such as mesenchymal stromal cells, reaching up to 25–30 μm in size [[14]Ge J Guo L Wang S Zhang Y Cai T Zhao RCH et al.The Size of Mesenchymal Stem Cells is a Significant Cause of Vascular Obstructions and Stroke.Stem Cell Reviews and Reports. 2014; 10: 295-303Crossref PubMed Scopus (154) Google Scholar]. Some cellular medicines are delivered as aggregates that may contain hundreds or thousands of cells, typically varying in size from approximately 50 µm to 250 µm. In addition, cells naturally shed particulate matter through cellular processes such as exocytosis, via release of EVs when proliferating and when reacting to their micro-environment or dying (apoptotic bodies) [15Bauer N Wilsch-Bräuninger M J Karbanová Fonseca AV Strauss D Freund D et al.Haematopoietic stem cell differentiation promotes the release of prominin-1/CD133-containing membrane vesicles–a role of the endocytic-exocytic pathway.EMBO Mol Med. 2011; 3: 398-409Crossref PubMed Scopus (86) Google Scholar, 16Kim SJ Moon GJ Cho YH Kang HY Hyung NK Kim D et al.Circulating Mesenchymal Stem Cells Microparticles in Patients with Cerebrovascular Disease.PLoS One. 2012; 7: e37036Crossref PubMed Scopus (40) Google Scholar, 17Strassburg S Hodson NW Hill PI Richardson SM Hoyland JA. Bi-Directional Exchange of Membrane Components Occurs during Co-Culture of Mesenchymal Stem Cells and Nucleus Pulposus Cells.PLoS One. 2012; 7: 33739Crossref PubMed Scopus (65) Google Scholar, 18Jaiswal R Sedger LM. Intercellular Vesicular Transfer by Exosomes, Microparticles and Oncosomes—Implications for Cancer Biology and Treatments.Frontiers in Oncology. 2019; 9: 125Crossref PubMed Scopus (61) Google Scholar]. Gene therapies based on small active particles such as LNPs (LNPs, EVs and viruses broadly range between 20 nm and 800 nm in size) (United States Pharmacopeia [USP] <1046>) and some viruses are oblong or irregular in shape. This poses a challenge when enumerating visible and subvisible particles per milliliter for release testing (European Pharmacopoeia 2.9.19, USP <788>). Cells and cellular material are considered particulate matter under current guidance, raising issues as to how terminology related to visual inspection methods and acceptance specifications should be defined in the release and quality control of a CGT. The authors opine that in certain advanced therapy drug types like LNPs and micro-volume gene therapies, potency and safety may be affected by intrinsic particles found in the manufacturing process. Particle generation from systems and consumables employed in the manufacturing process should be controlled during product development by suppliers in the manufacturing industry, such as those supplying single-use cell culture and liquid handling systems, sterile connectors, containment solutions in the cold chain and in-process analytics. Some sources of subvisible particles may be inherent to the cell therapy manufacturing process. One such example is the magnetic beads that are routinely used to isolate, sort and expand cell products, which potentially can become engulfed, attached or otherwise embedded in the finished product. Release assays are routinely performed to ensure that the final cell product has a defined level of purity, free from these exogenous materials (Table 1) [[19]Roddie C O'Reilly M Dias J Pinto A Vispute K Lowdell M. Manufacturing chimeric antigen receptor T cells: issues and challenges.Cytotherapy. 2019; 21: 327-340Abstract Full Text Full Text PDF PubMed Scopus (58) Google Scholar]. In an age of nanomaterials, coatings, laminations and other contaminant mitigation solutions, process designers should consider inorganic material shedding and accumulation utilizing applicable standards supplemented with analyses regarding the impact from the cold chain and freeze–thaw processes widely practiced with current CGT drug products [20Timmons CL Liu CY Merkle S Yuen Liu C Mitigation via New Glass Vial Particulate Generation Mechanisms during Bulk Filling and Particulate Generation Mechanisms during Bulk Filling and Mitigation via New Glass Vial.Particulate Generation Mechanisms during Bulk Filling and Mitigation via New Glass Vial. 2017; 71: 379-392Google Scholar, 21Anger S Begat C Crnko V Fantozzi GM Farach W Fitzpatrick S et al.Points to Consider: Best Practices to Identify Particle Entry Routes along the Manufacturing Process for Parenteral Formulations.PDA Journal of Pharmaceutical Science and Technology. 2019; 73: 635-647Crossref PubMed Scopus (1) Google Scholar, 22Halamoda-Kenzaoui B Ceridono M Colpo P Valsesia A Urbán P Ojea-Jiménez I et al.Dispersion Behaviour of Silica Nanoparticles in Biological Media and Its Influence on Cellular Uptake.PLoS One. 2015; 10e0141593Crossref PubMed Scopus (46) Google Scholar, 23Leclerc L Rima W Boudard D Pourchez J Forest V Bin V et al.Size of submicrometric and nanometric particles affect cellular uptake and biological activity of macrophages in vitro.Inhal Toxicol. 2012; 24: 580-588Crossref PubMed Scopus (32) Google Scholar, 24Adjei IM Sharma B Labhasetwar V. Nanoparticles: cellular uptake and cytotoxicity.Adv Exp Med Biol. 2014; 811: 74-91Google Scholar]. In addition, the effects of cell or gene delivery particle aggregation on CGT products have been investigated and may vary significantly depending on the product [[25]Li R Yu G Azarin SM Hubel A Freezing Responses in DMSO-Based Cryopreservation of Human iPS Cells: Aggregates Versus Single Cells.Tissue Eng Part C Methods. 2018; 24: 289-299Crossref PubMed Scopus (29) Google Scholar,[26]Xu X Liu Y Cui Z Wei Y Zhang L. Effects of osmotic and cold shock on adherent human mesenchymal stem cells during cryopreservation.Journal of Biotechnology. 2012; 162: 224-231Crossref PubMed Scopus (34) Google Scholar]. Although efforts are made to prevent or reduce aggregation that impacts therapeutic efficacy or manufacturing, it is unclear how to best approach cell or gene particle aggregates in the context of particulate testing for product release. Likewise, the impact of detection and safety limits on drug product stability and efficacy should be considered. Guidance and subsequent standardization of such limits will be important in the translation and regulation of CGTs specifically. Combination CGT–medical device products are leading to real-world particulate issues. For example, implanted medical devices utilized to encapsulate cells may contain inorganic particulates from the device manufacturing process. Other particulate challenges arise when creating organic particulates as therapeutic EVs, aggregated protein or inorganic–organic precipitate. The presence of such particulates may be relevant to clinical efficacy, such as in the case of tissue-engineered products, further complicating acceptance criteria and specifications for these types of therapeutics. Several compendial biocompatibility tests defined in International Organization for Standardization 10993 involve assessment of extractions using polar and non-polar solvents. During a routine extraction process, the technician observed that a couple of particles were present in the extract and noted this in the report. The test was performed per protocol and satisfied acceptance criteria, resulting in a pass. A regulatory reviewer who had requested the full report questioned the origin and type of particulate. Despite a standard memo, generated years earlier, indicating that sample extraction was not performed in a cleanroom environment and therefore particulate contamination was not uncommon, the regulator insisted that the company perform a root cause analysis. This required a repeat of the test under cleanroom conditions, with the source of the particulate being identified as personal protective equipment worn by test technicians Biologics such as antibodies, cytokines and receptor decoy therapies emerged and expanded rapidly by leveraging small-molecule process development and applied learning of protein-based therapeutics utilizing similar master cell banks and bioreactor production methods. Today's CGT growth encompasses multiple product types with numerous production methods that rely on production processes firmly rooted in research methods, lacking the benefit of having been previously translated to manufacturing using Good Manufacturing Practice standards for consistent execution and analysis. Figure 1 illustrates one of these products, highlighting possible particulate generation and elimination points in a generalized cell therapy manufacturing process. How do we, as an industry heavily reliant on single-use systems, define the significance of particulate matter in our therapies? Upscaling technologies such as bioreactors pose new questions with respect to potential system-specific intrinsic particulates (e.g., dissolvable micro-carriers or particles shed by filter cartridges). The requirement to maintain sterility throughout manufacturing, from collection to administration, creates unique challenges since typical methods used to sterilize traditional final drug products cause harm to the living and fragile drug products that define CGTs. Similarly, purification strategies such as in-line filtration methods pose challenges of particle shedding, as sterile filtration requires a polymer membrane that can also shed into the “clean” fluid path. Sterile connectors that puncture, create welds or require tear-off films may also contribute to particulate accumulation, but additional data are required to confirm this. Although particulates introduced from single-use systems can be filtered out of molecule-sized products, this cannot be as easily accomplished for many CGTs, and whereas advanced filtration methods may work for some, the challenge lies in defining and isolating a clean cell product that is free of intrinsic particles. How can such release criteria and limits be standardized with such vastly different production processes employed? Recently revised recommendations from the Bio-Process Systems Alliance regarding particulates in single-use systems provide helpful guidance [[27]Bio-Process Systems Alliance. BPSA 2020 recommendations For Testing, Evaluation, and Control of Particulates From Single-Use Process Equipment https://www.scribd.com/document/486229412/BPSA-2020-Recommendations-for-Testing-Evaluation-and-Control-of-Particulates-from-Single-Use-Process-Equipment (accessed June 24, 2022).Google Scholar], and task groups within ASTM Committee E55 are currently working on new standards. Running a process cycle with purified water or buffer alone can aid in the determination of sources of particulates released during processing, and single-use systems may benefit from flushing prior to application, especially components like filter cartridges. Importantly, risk scenarios vary widely, as small batch sizes and single lots can pose a challenge. For example, the production of 20 000 doses (1 mL) of a vaccine using a 20-L single-use bag with 20 visible particles yields a potential increase of only 0.1% in the rejection rate during a visual inspection. However, application of a small single-use bag with “only” a few visible particles for a CGT drug product could result in 100% rejection of the drug product during a visual inspection. The source, material, size and quantity of foreign particulate matter will impact human health based on the therapeutic route of administration. Micro-volume injections in the eye or central nervous system are likely to pose increased risks from particulates due to the nature of the therapy [[28]Dounce SM Laskina O Goldberg RA. Particulate matter from syringes used for intravitreal injections.Retina. 2021; 41: 827-833Crossref PubMed Scopus (4) Google Scholar], whereas particles contained in infusates administered into the vasculature pose an embolic risk [[11]Perez M Maiguy-Foinard A Barthélémy C Décaudin B Odou P. Particulate Matter in Injectable Drugs: Evaluation of Risks to Patients.Pharmaceutical Technology in Hospital Pharmacy. 2016; 1: 91-103Crossref Google Scholar]. Potency of cell-based implants may not be affected by inherent particle generation, but the quality of implanted cells may be adversely affected by particles potentially embedded during the additive manufacturing process, as in the case of tissue-engineered medicinal products. Anti-fouling technologies such as nanomaterials, coatings and laminations could also negatively affect the immune privilege of implanted materials because of erosion or delamination over time, possibly shedding particles throughout the life span of the product. To the authors’ knowledge, both the impact of such long-term product decay and the methods used to assess the impact are not widely known in combination advanced therapy medicinal product drug–devices. Real-time detection of the type and quantity of particulates could enable small-scale fill and finish operations to quickly release products in a manner amenable to both product quality (e.g., size distribution of LNPs and weight distribution of viral particles) and patient safety (absence of large or aggregated particulates). Similar technologies could also be used to detect unacceptable particulates in single-cell and aggregate suspensions as well as implanted combination devices, where the therapy itself is considered a particulate. Publication of USP chapters <790> and <1790> and European Pharmacopoeia chapter 5.17.2 for visual inspection of final drug products has provided more clarity on current regulatory requirements (Table 3). However, many questions remain unanswered, including detection capabilities, training and how these requirements should be interpreted for individual CGTs. The International Conference on Harmonization (ICH) tripartite guidelines, specifically ICH Q5C, state that for stability of biotechnological/biological products, visual appearance of the product should be routinely assessed, which in the case of solutions and suspensions should be an assessment of color and opacity. When considering the use of light obscuration techniques described in USP <788>, the guidelines state that “applicants should consult the appropriate regulatory authorities on a case-by-case basis to determine guidance for testing” (ICH Q5C). This further highlights the lack of standardization with respect to how best to evaluate these parameters and how to overcome the complexity associated with classifying CGTs as drug therapeutics.Table 3Current regulatory guidance on particulates in injectables.Reference StandardDocument TitlePh. Eur 2.9.20Particulate contamination: visible particlesPh. Eur 2.9.19Particulate contamination: subvisible particlesPh. Eur 5.17.2Recommendations on testing of particulate contamination: visible particles.USP <1>Injections and implanted drug productsUSP <788>Particulate matter in injectionsUSP <789>Particulate matter in ophthalmicsUSP <790>Visual particulates in injectionsUSP <1788>Particulate matter in injections and ophthalmic solutionsUSP <1790>Visual inspection of injectionsUSP <1044>Cryopreservation of cellsISO 23565ISO/TS 23565:2021—biotechnology—bioprocessing—general requirements and considerations for equipment systems used in the manufacturing of cells for therapeutic use; section referencing particulates (7.3.4)USP outlines documentary standards relied on by the FDA to ensure safety and quality of food and drugs. Ph. Eur is a single reference work for the quality control of medicines. The official standards it contains provide a scientific basis for quality control during the entire life cycle of a product.FDA, Food and Drug Administration; ISO, International Organization for Standardization; ISO/TS, International Organization for Standardization Technical Specification; Ph. Eur, European Pharmacopoeia. Open table in a new tab USP outlines documentary standards relied on by the FDA to ensure safety and quality of food and drugs. Ph. Eur is a single reference work for the quality control of medicines. The official standards it contains provide a scientific basis for quality control during the entire life cycle of a product. FDA, Food and Drug Administration; ISO, International Organization for Standardization; ISO/TS, International Organization for Standardization Technical Specification; Ph. Eur, European Pharmacopoeia. Challenges posed to therapy developers during manufacture are currently therapy-specific. Flow cytometry, other light obscuration technologies and optical systems have been used successfully to provide in-line, near real-time particle data in research [29Saggu M Patel AR Koulis T. A Random Forest Approach for Counting Silicone Oil Droplets and Protein Particles in Antibody Formulations Using Flow Microscopy.Pharmaceutical Research. 2016; 34: 479-491Crossref PubMed Scopus (29) Google Scholar, 30Calderon CP Daniels AL Randolph TW. Deep Convolutional Neural Network Analysis of Flow Imaging Microscopy Data to Classify Subvisible Particles in Protein Formulations.Journal of Pharmaceutical Sciences. 2018; 107: 999-1008Abstract Full Text Full Text PDF PubMed Scopus (48) Google Scholar, 31Rech J Fradkin A Krueger A Kraft C Paskiet D. Evaluation of Particle Techniques for the Characterization of Subvisible Particles From Elastomeric Closure Components.Journal of Pharmaceutical Sciences. 2020; 109: 1725-1735Abstract Full Text Full Text PDF PubMed Scopus (2) Google Scholar]. Some of these existing technologies should be leveraged into equipment and processes that enable on-demand, batch-oriented cell products to be manufactured cost-effectively. In addition, there has been significant movement toward the development of automatic inspection systems; however, significant challenges remain regarding how best to employ these systems with respect to parameters for quantification and validation. Contemporary automated cell and particle counting instrumentation relies heavily on software for determination of singlet, doublet and aggregated particles. Artificial intelligence is poised to dominate the discriminatory methods for determining particle size, distribution and type as detection and characterization methods improve. Technologies such as photometric mass determination combined with high-speed image processing may offer a significant improvement over pure human visual observation or simplistic light obscuration. A concerted effort to improve these technologies to permit discrimination between intended drug product components and contaminating particulates is required. Because of a lack of consensus particulate standards for CGTs or the medical devices used to deliver them, the industry has leveraged existing standards intended for parenteral drug products such as injectables for intravenous or intrathecal delivery (e.g., USP <787>, USP <788>) (Table 3). However, these standards were not designed to establish acceptance limits for CGTs or delivery into other anatomical sites. To develop new or updated standards, the industry should identify the challenges faced, including:(i)>What type of particulates need to be measured?(ii)What size and quantity of particulates are acceptable based on dose ranges, route of administration and therapeutic mechanism of action?(iii)How is this affected by the material or source of the particles?(iv)How does the anatomical delivery site affect acceptance limits?(v)What are the acceptable final release assays for particulate testing of the final drug product?(vi)What are the potential implications to patient safety?(vii)How should we measure different particulates, particularly within cell suspensions? CGT manufacturers and their suppliers need to identify and implement mitigations to limit particulates throughout the manufacturing process so that finished products can meet these future standards. Limitations exist around current drug purification processes, as they create challenging physical and environmental requirements for CGTs that need to maintain potency and, ultimately, clinical efficacy. Many CGTs inherently involve delivery of particulates into the patient, including cells and cellular aggregates in suspension. Can pre-clinical testing, including biocompatibility assessments and in vivo safety studies, be utilized to establish the safety of these products in a manner similar to that of verification and validation of medical devices? Consistent with the fundamental philosophy of medical product development, a quality risk management approach (ICH Q9) should take a central role in determining risks associated with particulates while balancing the benefit to patients. Risks associated with these emerging therapeutics and manufacturing paradigms are not always clear, and we need to work together in an open and transparent way to identify best practices in particulate prevention and assessment to ensure CGTs get to patients in a safe and timely manner. Although efforts are underway to establish advanced therapy-specific standards and guidance (Alliance for Regenerative Medicine Standards Coordinating Body), there is a lack of consensus in current guidelines, largely based on existing non-specific standards applied to legacy processes borne of research. Product-specific requirements and route of administration should guide product development and manufacturing process optimization based on specific particulate-related quality attributes. Related acceptance limits should consider the clinical administration procedure, number of injections/implants, total dose and likelihood of inadvertent delivery into vascular or intrathecal structures as well as particulate materials, size and quantity. To uncover the potential challenges that particles pose to CGTs, we, as an industry, need to openly and willingly share our challenges so that the entire community can learn and improve. Without direct feedback from stakeholders and the regulatory community, increasing approvals of advanced therapies are at risk.
更多查看译文
AI 理解论文
溯源树
样例
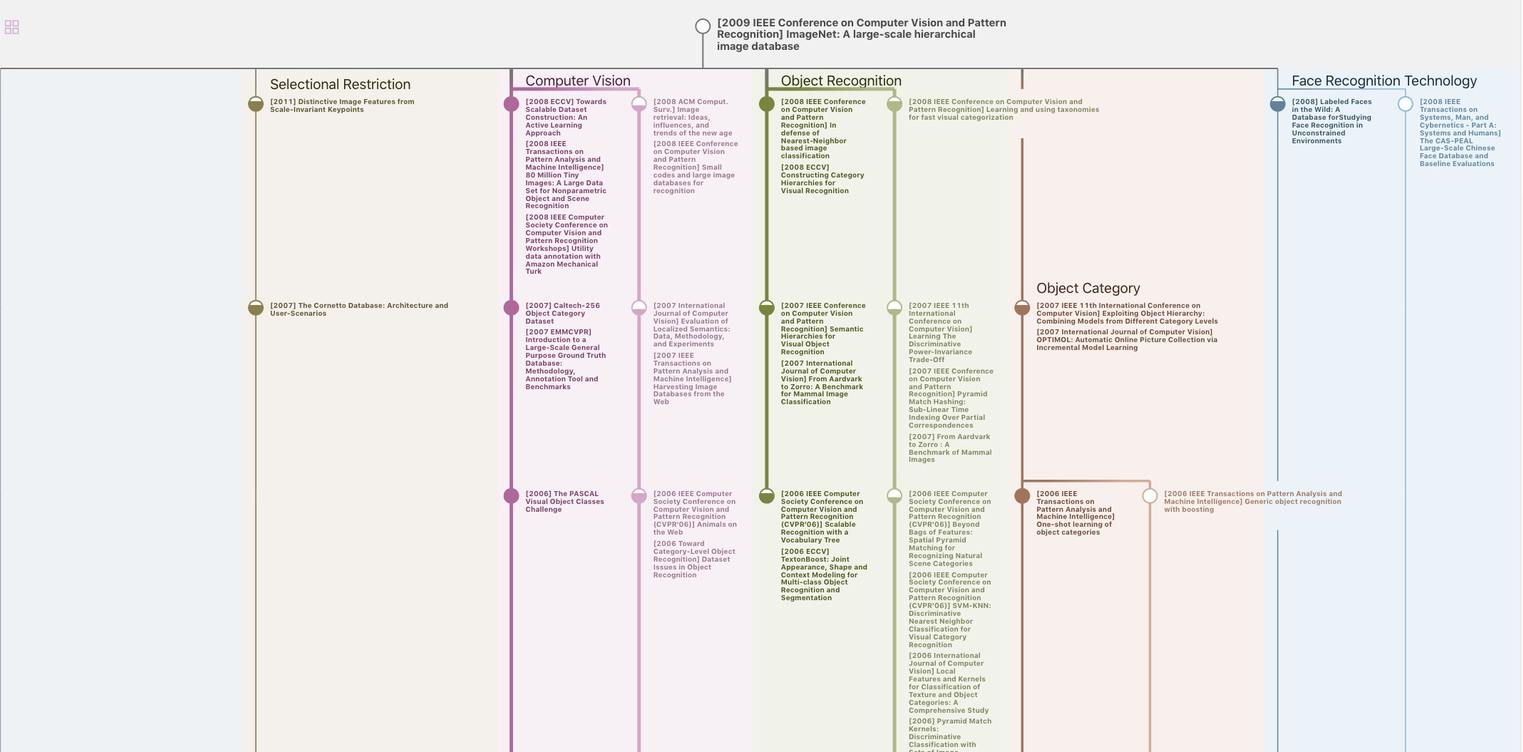
生成溯源树,研究论文发展脉络
Chat Paper
正在生成论文摘要