mRNA cytidine N4‐acetylation by NAT10 modulates lipid utilization in breast cancer
Clinical and Translational Discovery(2022)
摘要
When chemical modifications are deposited to mRNA molecules, they get the chance to alter the mRNA fates, including pre-mRNA splicing, mRNA transportation, mRNA stability, mRNA structure and translation efficiency.1 N4-acetylcytidine (ac4C) modification is the most recently discovered mRNA modification with the main function to increase mRNA stability and promote translation efficiency by altering intramolecular hydrogen bond and enhancing cytidine-guanine base pairing between the mRNA-tRNA duplex.2, 3 Deregulation of RNA modifications (more commonly called ‘epitranscriptome’) may contribute to pathogenesis. Cancer cells seem to be specifically addicted to these epitranscriptome modifying enzymes to sustain survival and gain proliferation competence.4 While N⁶-Methyladenosine (m6A) is the most characterized internal mRNA modification, the physiological and pathological relevance of the epitranscriptome beyond m6A, including ac4C modification, remains largely unknown. In this issue of Clinical and Translational Medicine, Dalhat et al. revealed the importance of RNA cytidine acetyltransferase (NAT10)-mediated ac4C modification in regulating the fatty acid metabolism in breast cancer5. The authors found that NAT10, the main enzyme responsible for RNA ac4C deposition was up-regulated in around 92% of human cancers, which hinted at the importance of NAT10-ac4C in carcinogenesis. This study started from Hela and MCF7 cells, two solid tumor cell lines with the highest NAT10 mRNA expression. Importantly, knockdown of NAT10 by siRNA led to the reduction of overall ac4C level. Coincidentally, the decrease of cell viability, induction of cell apoptosis and deficiency in mitochondrial biogenesis were also observed in the NAT10 knockdown cells. Mechanistically, fatty acid metabolic genes including ELOVL6, ACSL1, ACSL3, ACSL4, ACADSB and ACAT1 were down-regulated after NAT10 knockdown in MCF7 cells. Immunoprecipitation and quantitative reverse transcription PCR of acetylated RNA (acRIP-qPCR) assays confirmed the existence of ac4C modification in these mRNA transcripts. mRNA stability assay further showed that reduction of ac4C decreased mRNA half-life of these transcripts (Figure 1A). Since the fatty acid metabolism genes responsible for lipid droplet formation were significantly repressed after NAT10 knockdown, the authors hypothesized that cancer cells with NAT10 knockdown would have a disadvantage in regulating the turnover of fatty acid. To prove this, the authors performed an exogenous palmitate uptake assay. Palmitate (PA) was the most common saturated long-chain fatty acid found in animals. In cells, palmitate would firstly be processed to long-chain fatty acyl-CoA and then go through β-oxidation to produce acetyl-CoA in mitochondria or be elongated to very long-chain fatty acids, subsequently form triglycerides (TAGs) and lipid droplets in the cytoplasm. In palmitate uptake assay, the authors observed a noticeable accumulation of TAGs and lipids by Oil Red O staining in PA-siControl cells but not in PA-siNAT10 or vehicle-treated cells. Lipid profile analysis and BODIPY probe-based lipid droplet detection also suggested that overall lipid context, TAGs and lipid droplet formation were decreased in PA-siNAT10 cells compared with PA-siControl cells. Importantly, mRNA expressions of pivotal lipid accumulation genes including SREBF1, SRFBF2, LXRA and LXRB were suppressed in PA-siNAT10 cells. Moreover, NAT10 knockdown led to a ∼30% reduction of cell viability upon exogenous palmitate stimulus. These findings suggested that breast cancer cells with NAT10 depletion would lead to a deficiency in regulating fatty acid metabolism and lipid accumulation. More interestingly, the authors found that the mRNA expression of NAT10 drastically increased upon an exogenous palmitate stimulus, which suggests the importance of NAT10-ac4C dependent mechanism in the process of lipid accumulation driven by palmitate. The authors further performed acRIP-sequencing in palmitate-treated cells to profile the global mRNA acetylome. Importantly, they identified that 96% of fatty acid metabolic genes were enriched by ac4C-pulldown. Consistent with previous results, the authors found that the acRIP peaks of NAT10-ac4C target genes were decreased in PA-siNAT10 cells compared with PA-siControl cells. For these ac4C target genes, the result of the mRNA half-life assay also suggested that mRNA stability conferred by NAT10-ac4C seems to be more prominent when cells were undergoing exogenous palmitate stimulus. Remarkably, 496 of 2279 lipids were significantly altered after NAT10 knockdown according to the untargeted lipidomic profiling. Enriched pathways of down-regulated lipids included β-oxidation of very long chain fatty acid, fatty acid biosynthesis and ketone body metabolism. Surprisingly, acetyl-CoA, the key node of metabolism and also the substrate to support NAT10 mediated RNA acetylation, was remarkably reduced after NAT10 knockdown. The authors assumed such changes might be caused by the deficiency in fatty-acid utilization after NAT10 knockdown. ac4C reduction on ACSL1, ACSL3 and ACSL4 impaired their expression and further repressed the transformation of long-chain fatty acid to fatty acyl-CoA. Moreover, repressed expression of ac4C downstream gene ACADSB and ACAT1 constituted a hindrance to the β-oxidation of acyl-CoA in mitochondria, which was one of the major biosynthesis ways for cells to produce acetyl-CoA and energy (Figure 1B). Overall, Dalhat et al. showed that high expression of NAT10 in breast cancer cells confers the cancer cells a stronger ability in lipid utilization and fatty acid metabolism. Inhibition of NAT10 exposed the vulnerability of breast cancer cells and might be a potential target for cancer treatment. Nevertheless, several outstanding questions require further detailed investigations. Although 92% of cancers exhibit NAT10 overexpression, the target genes of NAT10-ac4C vary a lot in different types of cancer, which is similar to the situation of m6A in different cancers.4 Why these RNA modifying enzymes including NAT10 are universally needed in tumors while the modified mRNA targets are so distinct in different cancers remain unclear. Another question is the multi-substrate specificity of non-m6A epitranscriptome modifications. ac4C and also several other non-m6A epitranscriptome modifications depend on tRNA modification machinery.6 That means when targeting NAT10, not only mRNA but also tRNA and rRNA ac4C would be suppressed. Carefully designed experiments are necessary to exclude the possibility that the phenotypes are not contributed by non-mRNA ac4C inhibition, especially when the differential expressions of the putative mRNA ac4C target genes are subtle. Finally, the effort for pharmaceutically targeting NAT10-ac4C should not be abandoned; especially there are already some highly specific NAT10 binding molecules available. Although their efficacy for acetyltransferase activity inhibition seems to be weak.7, 8 Learn from the success of small molecular inhibitor development for m6A modification,9 investigators are expected to develop potent molecule for NAT10-ac4C inhibition in a foreseeable future. Mengjie Wu and Chun-Ming Wong contributed to the preparation and collection of original literatures and figures and the writing and editing of manuscript. Mengjie Wu and Chun-Ming Wong were responsible for the structural designs, scientific quality and writing. Not applicable. Not applicable. The authors declare no conflict of interest. Not applicable. Data sharing is not applicable to this article as no new data were created or analyzed in this study.
更多查看译文
关键词
nat10 modulates lipid utilization,mrna,breast cancer
AI 理解论文
溯源树
样例
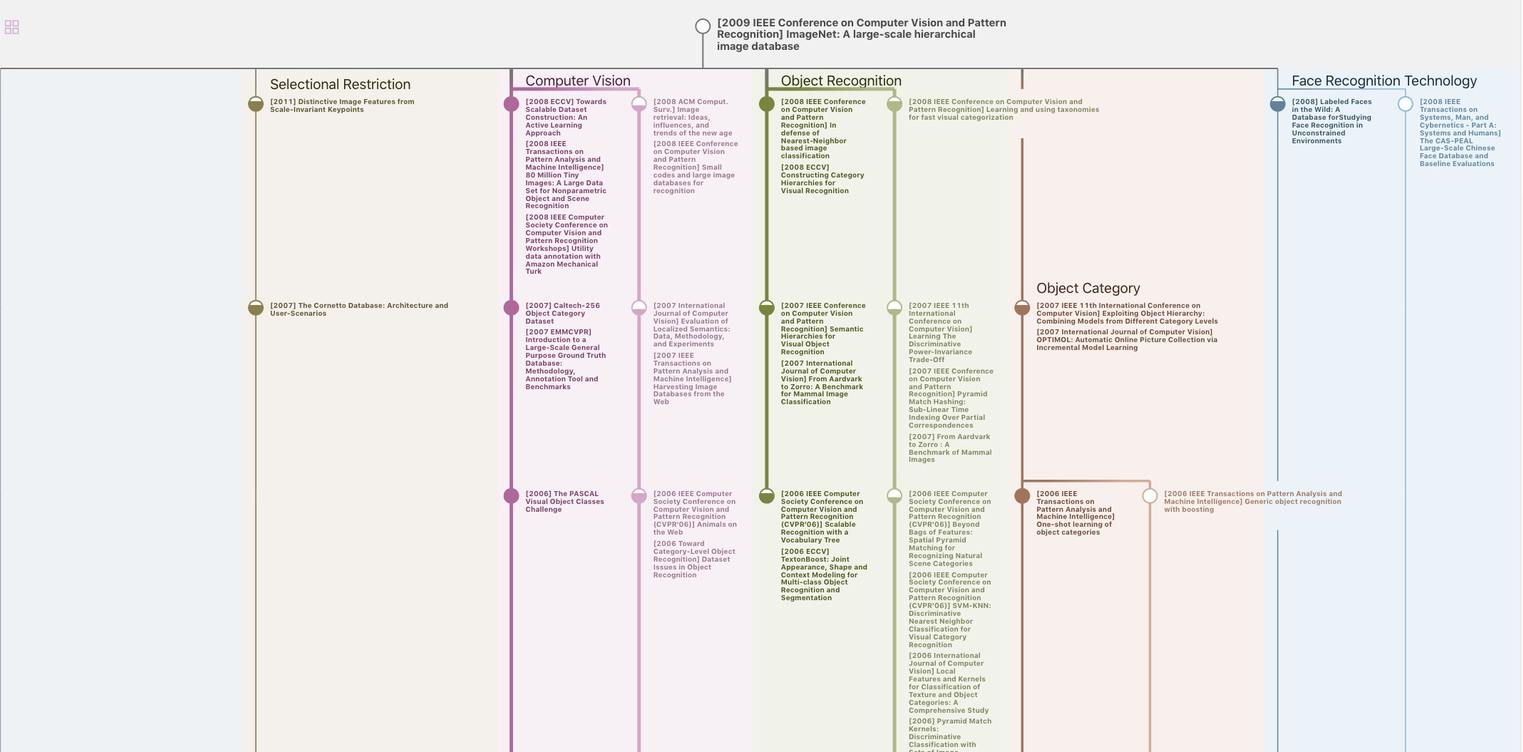
生成溯源树,研究论文发展脉络
Chat Paper
正在生成论文摘要