Sex chromosome evolution: The remarkable diversity in the evolutionary rates and mechanisms.
Journal of evolutionary biology(2022)
摘要
In this editorial letter, we summarize the current challenges in the field of sex chromosome evolution and introduce the 17 manuscripts which together provide insights into the diversity of sex chromosome evolutionary rates and mechanisms. Across the tree of life, many species with separate sexes have evolved sex chromosomes, on which (master) sex-determining genes are located to determine the sex of an individual (Bachtrog et al., 2014; Beukeboom & Perrin, 2014). Sex-linked inheritance makes sex chromosomes a hotspot for sex-specific and sexual selection, and independently evolved sex chromosomes show convergent evolutionary and genomic characteristics (Ellison & Bachtrog, 2019; Furman et al., 2020; Hughes & Rozen, 2012; Ming et al., 2011). In different species across eukaryotes, sex-limited chromosomes (i.e. Y or W chromosomes) have evolved to exhibit a wide range of divergence from their homologous X or Z chromosomes (Ashman et al., 2014; Furman et al., 2020; Wright et al., 2016). The level of degeneration of sex chromosomes is highly lineage-specific. Mammals, most birds and insects, and several lineages of reptiles, amphibians and teleosts have highly degenerated Y or W chromosomes (i.e. a large proportion of gene loss, accumulation of repetitive sequences, enrichment of heterochromatin etc.). In contrast, sex chromosomes in many reptiles, fishes, amphibians and some dioecious angiosperm plants remain homomorphic (i.e. indistinguishable under the microscope) and little differentiated (i.e. both Z/X and W/Y chromosomes maintain a similar gene content), which is typically interpreted as representing early stages of sex chromosome evolution (Ashman et al., 2014; Bachtrog et al., 2014; Beukeboom & Perrin, 2014; Furman et al., 2020; Ma & Veltsos, 2021). This special issue explores and expands various aspects of the classical paradigm of sex chromosome evolution. We have a collection of 17 studies covering five major themes in sex chromosome evolution. Theme 1 is genomics and methodological advances, in particular focusing on early-stage sex chromosome evolution. The detection of the timing of recombination suppression between sex chromosomes and evolutionary strata is theme 2. Theme 3 is the study of sex chromosome divergence and differentiation with new insights about dosage compensation mechanisms. Theme 4 focuses on the detection of (master) sex-determining genes and the turnover of sex chromosomes in (mostly) non-model organisms. Finally, theme 5 is the role of ecological factors in sex chromosome evolution. Both WGS and RAD-seq approaches can provide different insights into sex chromosome evolution, yet each has its own limitations. Using WGS to obtain high-quality sex chromosome assemblies remains quite expensive in organisms with large genomes (i.e. >2G bp). The sex-limited chromosome is notoriously difficult to assemble, even with current long-read sequencing technologies such as PacBio, Oxford Nanopore, Optical Mapping, and Hi-C sequencing (Sedlazeck et al., 2018; Yuan et al., 2017), due to the accumulation of heterochromatin and the presence of extensive low complexity, repetitive regions (Tomaszkiewicz et al., 2016). RAD-seq can generate thousands of genome-wide sequenced markers among many individuals at a reasonable cost, which works well to detect the presence of a XY or a ZW sex chromosome system even without a reference genome (Gamble et al., 2015). However, RAD-seq may fail to detect a small non-recombining region on sex chromosomes (i.e. proto-sex chromosomes), and it is difficult to compare distantly related species based on RAD-seq markers from fast-evolving non-coding regions (i.e. see Jeffries et al., 2018). Genomic sequencing alone can still fail to fully assemble sex chromosomes, thus complementary approaches such as cytogenetics can be helpful. Microdissection of sex chromosomes can greatly enrich the reads and coverage of sex chromosomes. Coupled with genome sequencing, it could help to detect genomic signatures of sex chromosomes. This approach is represented in this special issue by Pretto Gatto et al. (2022) who investigate the gene content and repeat regions on the sex chromosomes of the tocantins paradoxical frog. One challenge in the field is to identify signatures of divergence in homomorphic sex chromosomes. This is largely due to the substantial levels of sequence similarity between the X and the Y (or the Z and the W) chromosomes. Sometimes the methodologies used can lead to different conclusions for the same species (e.g. in guppy sex chromosome studies such as Wright et al., 2017; Bergero et al., 2019). In this issue, different analytical methods are applied to the same data to test their accuracy when investigating the divergence of young sex chromosomes in guppies. The authors conclude that the reference genome, as well as the mapping and filtering parameters can dramatically affect the results. They recommend the use of custom-made genome assemblies for mapping and to be cautious with the setting of parameters (Darolti et al., 2022). The evolution of sex chromosomes is hypothesized to involve a series of recombination cessation events at different time points, forming ‘evolutionary strata’ (Charlesworth, 2017; Charlesworth et al., 2005; Wilson & Makova, 2009). Those time points are commonly inferred from the level of sequence divergence of synonymous mutations (dS) or from phylogenetic relationships of gametologs across species within putative strata (Bergero et al., 2007; Carpentier et al., 2019; Krasovec et al., 2018; Stöck et al., 2011; Yazdi & Ellegren, 2014). However, drift and selection can affect sequences and introduce uncertainty. In this special issue, Zhang et al. (2022) apply two alternative phylogenetic approaches to estimate the time of recombination suppression between sex chromosomes: (i) the expected likelihood weight approach, which finds the most likely topology among a set of hypothetical topologies and (ii) the Bayesian approach that estimates the time of recombination cessation from specified calibration priors on a reference species topology. The authors further test the two methods on songbirds datasets, and both approaches yield similar estimates whilst outperforming the dS and maximum likelihood tree approaches. Furthermore, these two approaches worked better when analysing sequences from multiple species (Zhang et al., 2022). There is still limited understanding of the mechanisms of initiation and expansion of recombination suppression along sex chromosomes. Theory suggested that sexually antagonistic selection plays an important role in expanding the non-recombining region on the Y/W chromosome (Bachtrog, 2006; Bull, 1983; Charlesworth et al., 2005; Charlesworth & Crow, 1978; Fisher, 1931; Marais et al., 2005; Rice, 1987). However, empirical support for the role of sexually antagonistic variant is still very limited, and studies in anther-smut fungi have demonstrated that such genes are not necessary for recombination suppression (Bazzicalupo et al., 2019; Ma et al., 2020). Even without sexually antagonistic selection, recombination suppression between sex-related chromosomes initiated and further extended in a stepwise manner to generate evolutionary strata in the Microbotryum anther-smut fungi. In this special issue, Duhamel et al. (2022) significantly contribute to our understanding that various chromosomal rearrangements could bring two mating-type loci onto the same chromosome and of subsequent recombination suppression linking the mating-type loci. In total, they revealed that there were at least nine convergent events of mating-type locus linkage across the Microbotryum genus, making it an excellent model for studying convergent evolution of recombination cessation. In another included study, Filatov (2022) analyzes a recent shift of the pseudoautosomal boundary on the sex chromosomes in a dioecious plant Silene latifolia, offering an opportunity to study the processes operating during expansion of non-recombining regions. The overwhelming majority of viviparous mammals have a XX/XY sex chromosome system, and the Y chromosome is highly degenerated with a small fraction of functional genes due to the evolutionary consequences of long-term recombination suppression (Bachtrog, 2005; Cortez et al., 2014; Graves, 2006; Ohno, 2003). Thanks to model organisms such as humans and mice, we have a better understanding of the highly degenerated Y chromosomes. However, comparisons of the Y chromosomes of monkeys demonstrate that sex chromosome evolution had a different evolutionary trajectory in neotropical primates than in model mammals. In this special issue, Steinberg et al. (2022) indicated that the XY bivalent displays different meiotic behaviour in prophase I in the neotropical primates than it does in humans and that there are often multiple sex chromosome systems, originating from Y-autosome translocations in the neotropical primates when compared to the Old World monkeys. Similarly to all mammals who share homologous XX/XY sex chromosomes, all birds seem to share homologous ZZ/ZW sex chromosomes, which are highly degenerated in most lineages (Ashman et al., 2014; Handley et al., 2004; Stiglec et al., 2007; Wright et al., 2014). But even among them, sex chromosomes can acquire new material. Here, Sigeman et al. (2022) identify a novel neo-sex chromosome in an African warbler, where part of an autosome has been translocated to the Z chromosome. After translocation to the Z chromosome, the recombination suppression on the Z chromosome could gradually expand to part of or even to the whole autosomal region. The authors also find that the recombination of the translocated autosomal region in the studied species is only suppressed in a small region, compared with the complete suppression of the entire region in other Sylvioidea species, suggesting that the recombination continued along the region after the ancestral fusion event. This demonstrates that even within a group with highly degenerated Y or W chromosomes, such as non-human primates and songbirds, various lineages can still display considerable variation in the evolutionary trajectory of sex chromosomes. In contrast to the well-studied and highly degenerated mammalian and avian sex chromosomes, other lineages have sex chromosomes with little differentiation (Furman et al., 2020; Gammerdinger & Kocher, 2018; Ma & Veltsos, 2021). In these systems, detection of the divergence between sex chromosomes and of the variation within and across species is not always trivial (see review by Darolti et al., 2022). It is not uncommon to find intraspecific variation in sex chromosome differentiation (see Rodrigues et al., 2015; Jeffries et al., 2018; Ma et al., 2018 in various frogs). Evans et al. (2022) confirm that a 54.1 Mb sex-linked region on the W chromosome in the east Kenyan population of the Marsabit clawed frog is not sex-linked in the west Kenyan populations. This highlights a complex evolutionary trajectory of little differentiated, ‘young’ sex chromosomes and suggests that future work should focus on understanding the evolutionary dynamics of sex chromosomes and detection of sex-determining genes in various frog groups (see review by Ma & Veltsos, 2021). Sex chromosome degeneration may cause the evolution of dosage compensation (DC) mechanisms to restore the expression of the X (or Z) chromosome in the heterogametic sex to ancestral levels, or at least, to equalize between males and females to alleviate the potential detrimental phenotypic effects due to the loss of functional copies of genes on the Y (or W) chromosomes (Charlesworth, 1996; Darolti et al., 2019; Mank, 2009, 2013; Ohno, 1966). DC mechanisms can range from complete, covering the entire X (or Z) chromosomes, to partial which only act upon certain genes. The extent of DC varies dramatically across studied organisms even within closely related species (see reviews by Mank, 2009, 2013; Gu & Walters, 2017). In this special issue, several interesting findings are presented which provide further insights into this field. Parker et al. (2022) show that several Timema stick insect species with XX:XO sex determination have equal gene expression across the genome between sexes in somatic tissues (such as heads and legs), but dramatically reduce expression in males compared with females in reproductive tracts. The authors conclude there is complete DC in somatic tissues but a lack of DC in reproductive tissues. Their findings further highlight the importance of studying diverse tissues when assessing the extent of DC (also see Gu & Walters, 2017). Contrary to the standard gene expression comparison between sexes, DC can also be studied in the nucleolar organizing region (NOR). Montiel et al. (2022) reveale the NOR was sex-linked and that full DC upregulates the male X-NOR in a turtle with a XY sex chromosome system. This is not the case in another turtle species with a ZW system, where Z-NOR is silent in females but active in both Z chromosomes in males. The authors speculate that R2 retrotransposon retroelement disruption could contribute to W chromosome enlargement and facilitate recombination suppression between the Z and W chromosomes. Unlike the stable sex chromosomes of most mammals and birds, sex chromosomes have been found to go through frequent turnovers in some lineages of reptiles, amphibians and teleost fishes (Charlesworth, 2019; El Taher et al., 2021; Furman et al., 2020; Gamble et al., 2015; Jeffries et al., 2018; Ma & Veltsos, 2021; Vicoso, 2019). It remains an unresolved question why sex chromosome trajectories differ so dramatically across the tree of life. The first step towards understanding sex chromosome dynamics is to identify the sex-determining region. In this special issue, with available genome assembly, Jeffries et al. (2022) identify AmhY (a male-specific duplication of the Amh gene) as the candidate master sex-determining gene in a stickleback species. The authors also point out that this duplicate AmhY represented the second independent duplication and recruitment of Amh as a sex-determining gene, suggesting convergent evolution in two stickleback lineages. In a small minnow, the common creek chub, Meuser et al. (2022) find almost zero sex-linked markers with a RAD-seq approach, providing insights into the limitations of this technique for species with poorly differentiated sex chromosomes. Applying a similar RAD-seq pipeline, Keating et al. (2022) successfully identify male-specific markers in a banded gecko, suggesting the presence of a XX/XY sex chromosome system. The authors further demonstrated a sex chromosome turnover from an XY to another XY system in the banded geckos of the genus Coleonyx. Using pooled sequencing, Ansai et al. (2022) find that nine Oryzias fish species have XX/XY sex chromosome systems with several linkage groups used to determine sex in different species, whilst no sex chromosomes are found in four other investigated species. Their results suggest frequent turnovers in this lineage as well. Rovatsos et al. (2022) set out to provide insights into the phylogeny and the ancestral state of sex determination in squamate reptiles, which is still unresolved (reviewed by Kratochvíl, Gamble, et al., 2021). They identify a XX/XY sex chromosome system in a blind lizard, a member of a potential sister lineage to all other squamates, by comparative gene coverage analysis of a male and a female genome. The authors find that the X chromosome consists of genomic regions that have not been co-opted for this sex-linked function in other amniotes, indicating that it might be an apomorphy of blind lizards and hence uninformative in the quest for the reconstruction of the ancestral sex determination state of squamates. Sexual conflict has been considered to be an important driver of sex chromosome turnover (Van Doorn & Kirkpatrick, 2007, 2010). Meiotic drivers located on the sex chromosomes, typically on the X, generate intense intragenomic conflict, as they dramatically increase the proportion of daughters among the offspring by killing all Y-chromosome bearing sperm (Bachtrog, 2020; Jaenike, 2001). As meiotic drivers largely alter offspring sex ratio, they could further intensify sexual selection and sexual conflict within a population. Ma et al. (2022) hypothesize that this genetic conflict could contribute to sex chromosome turnover and tested this hypothesis in Drosophila affinis. In this species, the Y chromosome shows size variation, and males lacking a Y chromosome (XO) are fertile and males with the meiotically driving X and no Y produce only sons—effectively reversing the sex-ratio effect. With both empirical data and population genetic modelling, Ma et al. (2022) demonstrate that XO males were produced dramatically more via Y-chromosome disjunction, which was largely induced by meiotic drive and that O can prevent the spread of the equilibrium frequency of sex-ratio X chromosome but is unlikely to lead to the loss of the Y chromosome. The authors speculate that the O could lead to sex-chromosome turnover indirectly, for instance, an X-autosome fusion would also lead to a neo-Y chromosome segregating against the autosomal homologue of the neo-X. Furthermore, the neo-Y could complete the displacement of the ancestral Y because, unlike the O, it can accumulate male-beneficial mutations. The effect of selfish genetic elements, especially gamete killers like meiotic drivers that target the Y or W, on sex chromosome evolution remains poorly understood, and we anticipate further advances in this area as new genomic data becomes available. Sexually antagonistic selection and sexual selection are hypothesized to lead to recombination suppression between sex chromosomes, cause a faster evolutionary rate of sex-linked genes, affect gene content and also cause sex chromosome turnovers (Barton & Charlesworth, 1998; Charlesworth, 2017, 2021; Van Doorn & Kirkpatrick, 2007, 2010). Despite this widely accepted view, there is limited empirical evidence, such as evidence of sexually antagonistic genes driving sex chromosome recombination suppression and expansion. Are there more factors contributing to the process of sex chromosome differentiation? Meisel (2022) argues that sex-specific selection in different habitats caused by sex-specific vulnerability to different environmental factors or pathogens, could lead to niche partitioning or different dietary strategies between sexes, which might affect the dynamics of sex chromosome evolution. The author goes on to suggest that the evolution of sex determination can be affected by ecological factors, especially in species where the sex determination pathway is sensitive to temperature, such as in some reptiles, teleost fishes and other vertebrates. Furthermore, the author provided evidence that Y-linked alleles could affect pigmentation in ecologically relevant ways in poecilid fishes or affect body size by an interaction with environmental factors such as temperature. Studies on non-model organisms have identified sex chromosomes and sex-determining genes on a wide range of species, and many of them have found frequent turnovers either between XY and ZW systems or within XY or ZW systems. Future work should focus on identifying the underlying forces and genetic mechanisms driving sex chromosome turnovers. In addition, it should also focus on the importance of ecologically relevant selection pressure in sex chromosome turnovers (Meisel, 2022). Identifying how and what makes the sex-determining mechanisms so labile in certain lineages, but very stable in others, is still a challenge. Other than sexually antagonistic selection which has been hypothesized to drive recombination suppression between sex chromosomes, alternative forces or mechanisms are emerging (see Jay et al., 2022; Kratochvíl, Gamble, et al., 2021; Lenormand et al., 2020) and importantly need to be tested for support with wide empirical evidence. Genomic data are constantly collected from diverse taxa across the tree of life, and therefore, these resources need to be urgently updated and collated. This would be a great help when addressing related questions on the evolution of sex chromosomes and sex determination. Wen-Juan Ma: Conceptualization (equal); funding acquisition (equal); investigation (equal); methodology (equal); project administration (equal); resources (equal); validation (equal); writing – original draft (lead); writing – review and editing (equal). Michail Rovatsos: Conceptualization (equal); funding acquisition (equal); investigation (equal); methodology (equal); project administration (equal); resources (equal); validation (equal); writing – original draft (supporting); writing – review and editing (equal). We would like to thank all participants of the 2021 ESEB satellite symposium The remarkable diversity in the rate and mechanisms of sex chromosome evolution for sharing their research, thoughts and for the inspiring discussions and the exchange of ideas in a lively and welcoming atmosphere. We thank Lukáš Kratochvíl and Paris Veltsos for feedback and suggestions on previous versions of the manuscript. We also thank Luke Holman and another anonymous reviewer for their helpful comments. We thank all of the authors for contributing to this special issue. Special thanks also to Nicola Cook and Max Reuter for hosting this special issue and for all of the coordination and support in its preparation. We also thank Amy Wilson for proofreading the final version of the manuscript. This work is supported by Kansas IDeA Network of Biomedical Research Excellence (K-INBRE) postdoc grant P20GM103418 to W.-J. Ma and by the National Science Foundation, Division of Molecular and Cellular Bioscience CAREER grant 2047052 (to Robert L. Unckless). The authors declare that they have no conflicts of interest regarding this manuscript. The peer review history for this article is available at https://publons.com/publon/10.1111/jeb.14119.
更多查看译文
关键词
sex chromosome evolution,evolutionary rates
AI 理解论文
溯源树
样例
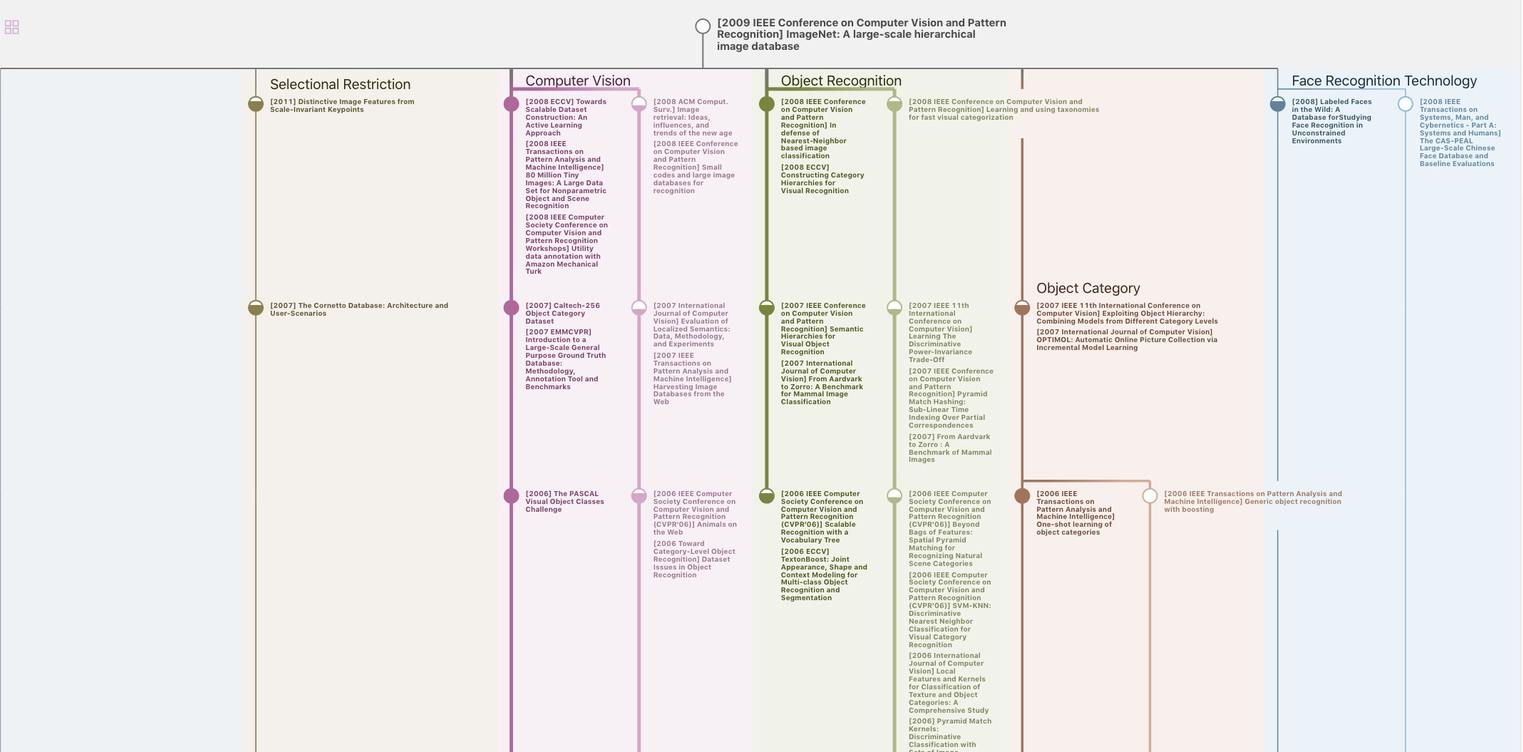
生成溯源树,研究论文发展脉络
Chat Paper
正在生成论文摘要