Species-specific stomatal ABA responses in juvenile ferns grown from spores
NEW PHYTOLOGIST(2023)
摘要
Stomatal pores in leaves control CO2 entry for photosynthesis and water loss via transpiration. Stomata open in response to light, low CO2 levels and high air humidity, and close in response to darkness, elevated CO2 concentration, low air and soil humidity. Stomatal closure responses are largely mediated by the stress hormone abscisic acid (ABA) which is produced in response to drought and reduced air humidity (Xiong & Zhu, 2003; McAdam et al., 2016b). ABA is perceived by ABA receptors from the PYR/PYL family that form a complex with PP2C phosphatases and suppress their activity (Ma et al., 2009; Melcher et al., 2009; Park et al., 2009). Suppression of PP2C activity allows the activation of the OST1 kinase that phosphorylates and activates the SLAC1 anion channel, triggering ABA-induced stomatal closure (Negi et al., 2008; Vahisalu et al., 2008, 2010; Fujii et al., 2009; Geiger et al., 2009; Lee et al., 2009). How the molecular mechanisms that coordinate stomatal responses to environmental change and ABA have evolved has intrigued stomatal biologists over the last decade. Lycophytes and ferns are one of the oldest plant groups with stomata (Clark et al., 2022). Ferns have been proposed to completely lack active ABA-induced stomatal closure and to close stomata via solely hydropassive mechanisms (Brodribb et al., 2009; Brodribb & McAdam, 2011; McAdam & Brodribb, 2012; Cardoso et al., 2019). At the same time, ABA-induced stomatal closure has been documented in several fern and lycophyte species (Ruszala et al., 2011; Cai et al., 2017; Hõrak et al., 2017; Wu et al., 2020). Several studies have also indicated that fern stomatal responses are species-specific and vary with growth conditions (Hõrak et al., 2017; Plackett et al., 2021). The species specificity may be explained by different numbers of ABA receptors and varying degrees of their ABA sensitivity in different species (Sun et al., 2019), and different degrees of activation of the SLAC1 anion channel via OST1 homologues of different fern or lycophyte species (Ruszala et al., 2011; McAdam et al., 2016a). The conditionality of fern stomatal responses may result from different lifetime growth conditions leading to, for example, differences in endogenous ABA levels (McAdam & Brodribb, 2012; Cardoso et al., 2019). Previous studies on fern stomatal physiology have often focused on rhizome-grown mature plants. Changes in environmental conditions experienced throughout the lifetime of the plant may have resulted in adaptations to local growth environment in such plants, potentially introducing legacy effects that affect their response to external cues. Growth conditions affected ABA responsiveness in the ferns Athyrium filix-femina and Dryopteris filix-mas (Hõrak et al., 2017), and priming of ABA responses was found in Ceratopteris richardii, where stomata became ABA responsive after pretreatment with ABA (Plackett et al., 2021). Here, we aimed to minimize priming and life history effects via analysis of nine fern species grown from spores under controlled growth conditions: Adiantum capillus-veneris, Adiantum polyphyllum, Cheilantes distans, Cheilantes farinosa, Cheilantes lanosa, Asplenium scolopendrium, Blechnum occidentale, Cyrtomium falcatum and Dryopteris sieboldii. We then analysed steady-state gas-exchange, stomatal density and ABA responsiveness in the juvenile leaves of these ferns (data available as Supporting Information Dataset S1) to resolve the physiological and anatomical controls on fern stomatal responsiveness to ABA. We germinated the spores under humid conditions and achieved gametophytes (Fig. 1a) that successfully fertilized and gave rise to sporophytes that could be analysed in the leaf chamber of a gas-exchange system (Fig. 1b; see also details in the Materials and Methods section). In line with previous studies on mature ferns (Hõrak et al., 2017; Xiong & Flexas, 2020; Cândido-Sobrinho et al., 2022), stomatal conductance (gs) and net photosynthesis rate (Anet) in juvenile ferns were generally low (Fig. 1c,d). gs varied sevenfold between the studied species, ranging from 20 to 150 mmol m−2 s−1 (Fig. 1c), whereas variation in Anet was even larger, ranging from 0.5 to 5 μmol m−2 s−1 (Fig. 1d). Ferns from the Cheilantes genus had the highest gs values in our study. There was a strong positive relationship between gs and Anet (Fig. 1e, R2 = 0.68, P = 0.004), similar to mature ferns (Kübarsepp et al., 2020) and across angiosperms (Wright et al., 2004). Ferns are known to have large and sparse stomata (Lima et al., 2019; Kübarsepp et al., 2020; Xiong & Flexas, 2020). In line with previous studies, we found low stomatal density in juvenile leaves of the studied species, with most values of c. 20 stomata mm−2. Dryopteris sieboldii had the highest stomatal density of c. 50 stomata mm−2 (Fig. 1f). In most species, stomatal index (the proportion of stomata from all epidermal cells) was c. 0.12 with a lower value observed in A. scolopendrium and a higher in D. sieboldii (Fig. 1g). Compared with typical stomatal density and index values in angiosperms, ferns strike out has having few large stomata (Kübarsepp et al., 2020). This might contribute to the sluggishness of their stomatal responses, as stomatal size and speed are negatively correlated (Drake et al., 2013; Kübarsepp et al., 2020). We found no significant relationship between stomatal density and gs (Fig. 1h), but in our sample, there was a linear positive relationship between stomatal density and Anet (R2 = 0.74, P = 0.002, Fig. 1i). These data suggest that in our experiments, Anet was indirectly affected by stomatal density, potentially via simultaneous adjustment of stomatal density and anatomical factors determining photosynthetic capacity, such as leaf thickness and mesophyll structure. To test whether juvenile ferns grown from spores under controlled conditions can respond to ABA, we spray-applied 10 μM ABA or mock solution (see details in the Materials and Methods section) and followed stomatal conductance in treated leaves for 1.5 h after the treatment (Figs 2a–i, S1). The low stomatal conductance of ferns was in some cases accompanied by large variation and fluctuations in gs (e.g. Fig. 2b,f), and in two Cheilantes species, mock treatment also affected gs (Fig. 2c,e). However, it was possible to clearly differentiate ABA-responsive and unresponsive fern species in our sample. External ABA triggered significant stomatal closure compared with mock treatment in C. distans, C. lanosa, B. occidentale and C. falcatum (Fig. 2c,e,g,h,j), whereas no difference between mock- and ABA-treated leaves was observed in A. capillus-veneris, A. polyphyllum, C. farinosa, A. scolopendrium and D. sieboldii (Fig. 2a,b,d,f,i,j). In ABA-responsive ferns, the responses were slow and relatively low in magnitude (Fig. 2c,e,g,h,j). This might explain why no ABA responses were previously found in shorter experiments (Cândido-Sobrinho et al., 2022). Ferns with relatively high initial gs tended to close stomata in response to ABA (Figs 1a, 2c,e,g,j), potentially suggesting that higher doses of ABA received through open stomata may account for the differences in ABA response between species. To account for differences in stomatal densities between species, we calculated normalized stomatal conductance (gs per million stomata, gsp) and analysed its relationship with ABA-induced stomatal closure. There was a significant positive linear relationship between gsp and ABA-induced change in gs (R2 = 0.65, P = 0.005, Fig. 2k) which was absent in mock-treated plants (R2 = 0.29, P = 0.08). Thus, it is possible that different ABA responses between fern species may be to some extent explained by different ABA doses that reach guard cells in ferns with different stomatal apertures and densities. Nevertheless, in our study, the fern C. falcatum that had one of the lowest gs values before treatment (Fig. 1c) and hence potentially low ABA uptake, showed a clear and relatively strong (c. 35%) ABA-specific stomatal closure, suggesting high ABA sensitivity of the ABA-signalling pathway in this species. Even the species that closed stomata in response to ABA had a slow response of variable, but mostly small magnitude, as documented before for mature ferns (Hõrak et al., 2017; Plackett et al., 2021). Thus, although some fern species can respond to ABA, the mechanisms underlying these responses, or their ABA sensitivity, are likely at least partly different from angiosperms. The reduced ABA sensitivity of ferns may be explained by lower ABA sensitivity of fern ABA receptors (Sun et al., 2019). While it is known that fern leaf ABA levels rise to a similar level as in angiosperms in response to drought stress (McAdam & Brodribb, 2012), fern guard cell ABA levels have not been measured. To understand the varying degrees of ABA responsiveness in diverse ferns, it is important to measure fern guard cell ABA levels under stress conditions and in response to exogenous ABA application and characterize the affinity of ABA receptors in respective species. In addition, the anatomical characteristics of fern stomata (large stomata with reduced mechanical advantage of epidermal cells over guard cells; Franks & Farquhar, 2007; Franks, 2013; Xiong & Flexas, 2020) may contribute to slow stomatal responses in ferns. Due to the lack of humidification in our growth room (see the Materials and Methods section), it is possible that the stomata in all studied species were primed for ABA responsiveness by the relatively dry indoor air (c. 50% relative humidity) as found before for C. richardii (Plackett et al., 2021). Even under these conditions, five of the nine studied species were completely insensitive to ABA, suggesting that while fern stomatal responses can be triggered conditionally in some species, ABA responsiveness in ferns is species-specific and absent in many species, in line with previously reported ABA insensitivity of some ferns (Brodribb & McAdam, 2011; Gong et al., 2021). As the ABA signalosome (Sun et al., 2020) and its role in stomatal responses (Clark et al., 2022) are considered an ancestral state present in the common ancestor of land plants, the absence of ABA responses in some ferns may be explained by secondary loss of ABA-response pathway components, or reduction in ABA sensitivity of these components. Variation in ABA sensitivity between ABA receptors is known to occur both within and between species (Sun et al., 2019), and it can be expected to translate into different stomatal ABA sensitivity. Hence, species-specificity of fern stomatal ABA responses is expected, although environmental conditions experienced throughout the lifetime likely affect both anatomical traits and physiology of stomatal responsiveness in ferns (Hõrak et al., 2017; Plackett et al., 2021). Indeed, conditionality of stomatal ABA responses dependent on relative air humidity has also been found in the angiosperm Arabidopsis (Pantin et al., 2013) and in multiple fern species (Hõrak et al., 2017; Plackett et al., 2021). Thus, as discussed also by Clark et al. (2022), priming of the stomatal closure pathway may be needed for efficient stomatal closure in ferns, and hence, depending on growth conditions, stomatal responses in ferns may sometimes remain undetected. In addition to the ABA receptors, downstream guard cell ABA-signalling components in angiosperms also include Ca2+-dependent protein kinases and reactive oxygen species (ROS; Munemasa et al., 2015). Both low relative air humidity and exogenous ABA that stimulated stomatal ABA responsiveness in the fern C. richardii also triggered transcriptional changes in genes encoding respective signalling components (Plackett et al., 2021). The authors propose that during sensitization of fern stomata with low humidity or exogenous ABA, the guard cell Ca2+, ROS and ABA-signalling cascades are primed to reduce the threshold necessary to trigger their activation, potentially explaining the conditional nature of fern stomatal responses. Priming of stomatal Ca2+-responsiveness by ABA has been proposed to occur also in angiosperms (Brandt et al., 2015), so potentiation of guard cell Ca2+-signalling may represent a conserved mechanism in stomatal responsiveness. Phylogenomic analyses suggest that orthologues of several components of the angiosperm stomatal aperture regulation network, such as SLAC1, OST1 and ABI1, were present in the common ancestor of land plants (Harris et al., 2020, 2022). However, later gene duplications in the tracheophyte lineage may have led to different functions evolving for these components, including different contributions to ABA-induced stomatal closure in ferns and angiosperms. Thus, it is important to functionally characterize the fern orthologues of angiosperm ABA-signalling pathway components to understand their role in fern physiology. Stomatal closure responses to elevated CO2 have often been discussed together with ABA responsiveness in ferns, implying similar underlying molecular mechanisms for these responses. Most studies have found that ferns close their stomata in response to elevated CO2, although the response is lower in magnitude and slower than in angiosperms (Franks & Britton-Harper, 2016; Hõrak et al., 2017; Lima et al., 2019; Kübarsepp et al., 2020; Wu et al., 2020). No response has been observed in a few cases (Brodribb et al., 2009; Brodribb & McAdam, 2013). While responses to elevated CO2 are indeed partly mediated via the ABA pathway in angiosperms (Merilo et al., 2013; Chater et al., 2015; Dittrich et al., 2019; Movahedi et al., 2021), and thus, possibly also in ferns, in the model angiosperm Arabidopsis thaliana, CO2-induced stomatal closure largely relies on the signalling module involving the HT1 and MPK12 kinases (Hashimoto et al., 2006; Hõrak et al., 2016; Jakobson et al., 2016; Takahashi et al., 2022; Yeh et al., 2023). An HT1 homologue is expressed in the stomata-bearing sporophyte of the moss Physcomitrium patens (Chater et al., 2013; O'Donoghue et al., 2013), but whether its function is conserved from mosses to angiosperms is unclear (Harris et al., 2020). Thus, analysis of fern HT1 pathway genes and their functional conservation will be important to understand the evolution of signalling pathways that control plant stomatal responses to changes in CO2 concentration. Given the stomata-specific role of the HT1-MPK12 pathway in A. thaliana, it can be a good indicator for evolution of stomatal CO2 responsiveness that is not confounded by pleiotropic effects that can be expected for ABA, a hormone with diverse roles in plants. Our study using ferns grown from spores under controlled conditions indicates that fern species-specific stomatal responsiveness is genetically determined and cannot be explained solely by different life history and growth conditions experienced over the plant lifetime. The mounting evidence on the variability of fern stomatal responses to ABA across species suggests that ferns constitute a diverse group in terms of their ABA responses. As sequencing and omic approaches become widely accessible, it will be possible to characterize the ABA- and CO2-response pathways in more fern species to better understand the evolution of stomatal responsiveness. Our study shows that there are ABA-insensitive species, and also that in the ABA-sensitive species, the responsiveness is often stronger in species with greater overall capacity for water loss (higher gs). This might indicate sensitization of stomatal response pathways in fern species with higher gs, as fine-tuning of water use can be particularly relevant for species with potentially greater physiological activity. We included nine fern species in our study: Adiantum capillus-veneris (University of Tartu Botanical Garden), Adiantum polyphyllum (University of Tartu Botanical Garden), Cheilantes distans (University of Tartu Botanical Garden), Cheilantes farinosa (University of Tartu Botanical Garden), Cheilantes lanosa (University of Tartu Botanical Garden), Asplenium scolopendrium (University of Tartu Botanical Garden), Blechnum occidentale (New Zealand), Cyrtomium falcatum (Estonian University of Life Sciences) and Dryopteris sieboldii (University of Tartu Botanical Garden). We grew the plants in a growth room (temperature 23°C, photoperiod 12 h : 12 h, moderate growth light level 50 μmol m−2 s−1 reflecting the conditions in the forest floor, and relative humidity was not controlled but was c. 50%) throughout the experiments. We sowed fern spores into 0.29 l (8 × 8 × 7 cm, length × width × height) plastic pots (Teku, Pöppelmann, Germany) with wet soil (commercial garden soil (N : P : K, 10 : 8 : 16; Kekkilä Group, Vantaa, Finland), and perlite in a 1 : 1 volume ratio) and incubated these in transparent plastic bags to preserve humidity and stimulate growth of gametophytes (Fig. 1a) and fertilization. When the first leaves of sporophytes emerged, we removed the plastic bags and transplanted individual sporophytes to separate pots for subsequent growth. After four to eight juvenile leaves had reached sufficient size to be analysed by a portable gas-exchange system (GFS-3000; Heinz Walz GmbH, Effeltrich, Germany), we carried out measurements of stomatal conductance (gs) and net photosynthesis (Anet) under the following conditions: 300 μmol m−2 s−1 light, 70% relative humidity, 400 μmol mol−1 CO2, cuvette temperature 25°C. Thereafter, we subjected each measured plant to either mock (0.01% surfactant Silwet L-77 in water) or ABA (10 μM ABA, 0.01% Silwet L-77 in water) treatment. Briefly, we removed the analysed leaf from the leaf cuvette, sprayed it with treatment solution, air-dried the leaf and inserted it back into the cuvette within c. 5 min, taking care to clamp the same part of the leaf into the cuvette as done before the treatment. We followed stomatal conductance of the treated leaf for 1.5 h to assess stomatal response to ABA. We calculated relative stomatal response curves with respect to pretreatment gs and relative and absolute changes in gs during the 90 min after treatment (change in gs in relative units or in mmol m−2 s−1 between gs at time point 0 and gs at 90 min after treatment). To characterize stomatal density and index, we captured dental silicone (Xantopren L blue; Kulzer Nordic AB, Helsingborg, Sweden) imprints from lower (abaxial) side of leaves and then applied nail varnish to derive transparent impressions as in Casson et al. (2009). We used a brightfield microscope (OBF 133C832, with digital camera ODC 832; KERN & SOHN GmbH, Balingen, Germany) and MicroscopeVIS2.0Pro software (KERN & SOHN GmbH) to capture images of 0.26 mm2 areas and quantified stomatal density (stomata mm−2) and index (proportion of stomata from all epidermal cells) with ImageJ (Schneider et al., 2012). Data were analysed with one-way ANOVA followed by a Tukey post hoc test, multiple t-tests with Bonferroni correction, or linear regression as indicated in the figure legends. OriginPro 2018 (OriginLab, Massachusetts, USA) was used for statistical analysis, and all effects were considered significant at P < 0.05. We thank Sten Mander from the University of Tartu Botanical Garden for providing fern spores for A. capillus-veneris, A. polyphyllum, C. distans, C. farinosa, C. lanosa, A. scolopendrium and D. sieboldii. This work was supported by the Estonian Research Council (grants PRG537 and PSG404) and the European Regional Development Fund (Centre of Excellence EcolChange). None declared. ÜN initiated the work with fern spores. HH conceived and designed the study. TW and HH collected data. TW and HH analysed data. ÜN contributed to data analyses. HH wrote the manuscript with TW and ÜN. The data that support the findings of this study are included as Dataset S1. Dataset S1 Experimental data supporting the findings of the study. Fig. S1 Stomatal abscisic acid responses in juvenile ferns in absolute units. Please note: Wiley is not responsible for the content or functionality of any Supporting Information supplied by the authors. Any queries (other than missing material) should be directed to the New Phytologist Central Office. Please note: The publisher is not responsible for the content or functionality of any supporting information supplied by the authors. Any queries (other than missing content) should be directed to the corresponding author for the article.
更多查看译文
关键词
abscisic acid (ABA) response,evolution of signalling pathways,ferns,guard cell signalling,stomatal physiology
AI 理解论文
溯源树
样例
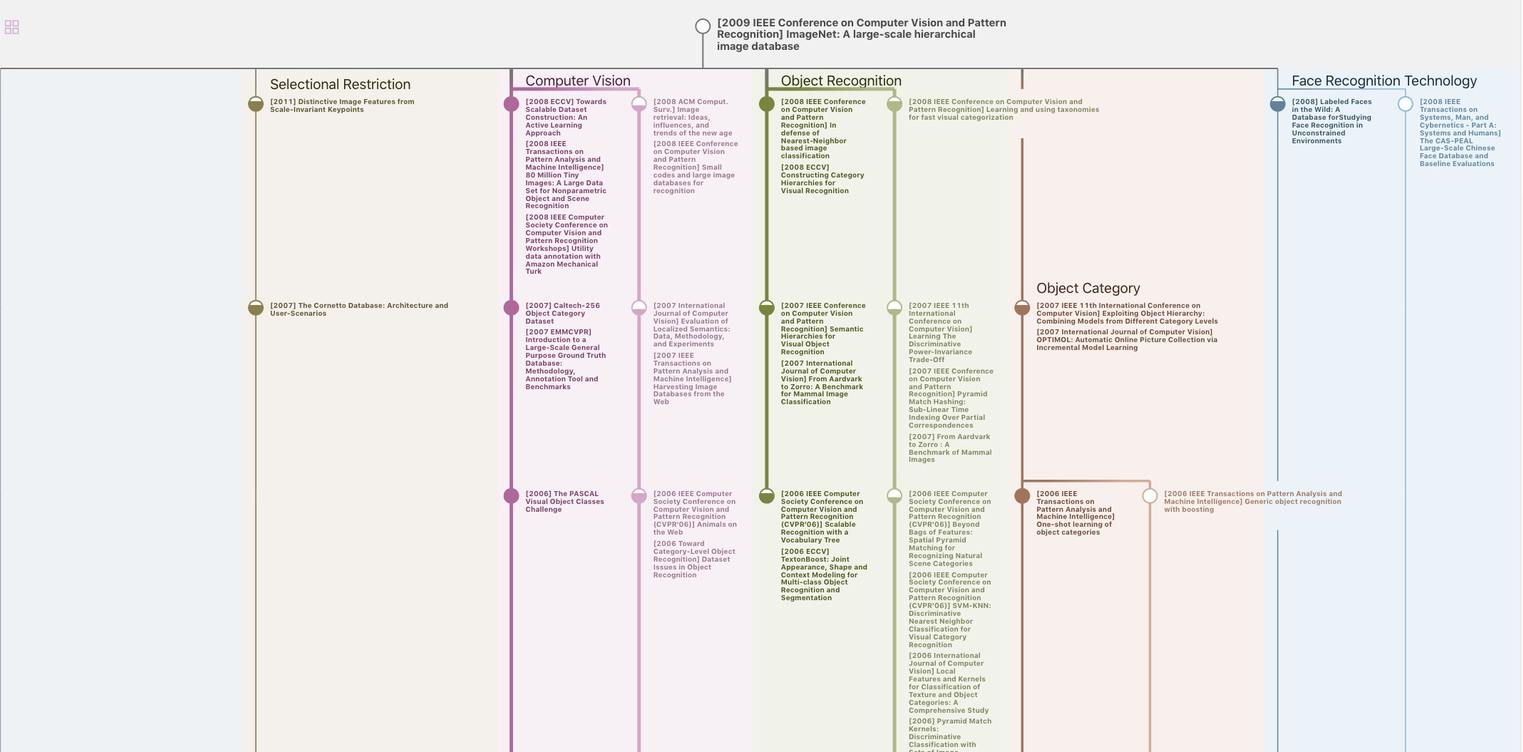
生成溯源树,研究论文发展脉络
Chat Paper
正在生成论文摘要