Design of high-monounsaturated fatty acid soybean seed oil using GmPDCTs knockout via a CRISPR-Cas9 system
Plant biotechnology journal(2023)
摘要
Soybeans (Glycine max (L.) Merr.) are critical resources for vegetable oil production for human and animal consumption as well as biofuel synthesis. Soybean oils are primarily composed of triacylglycerols (TAGs) with a certain mixture of fatty acid moieties. In general, the composition is approximately 62% polyunsaturated fatty acids (PUFAs), 23% monounsaturated fatty acids (MUFAs) and 15% saturated fatty acids (SFAs). The relatively high concentration of PUFAs linolenic acid (C18:3) and linoleic acid (C18:2) heavily contribute to oxidative rancidity, flavour reversion and short shelf-life of soybean oil (Warner and Gupta, 2005). In contrast, enhanced MUFAs oleic acid (C18:1) content in vegetable oils can produce significant health benefits, as well as improved oxidative stability, which is essential for both food usage and biodiesel and other renewable resource syntheses (Haslam et al., 2013; Knothe, 2005). Phosphatidylcholine:diacylglycerol cholinephosphotransferase (PDCT) serves as a catalyst for the interconversion of phosphatidylcholine (PC) and diacylglycerol (DAG). This, in turn enriches the PC-modified fatty acids in the DAG pool before TAG formation. Emerging studies revealed that a knockout or knockdown of PDCT genes enhances MUFAs levels while decreasing PUFAs among Arabidopsis (Lu et al., 2009), Crambe abyssinica (Guan et al., 2015) and Thlaspi arvense (Jarvis et al., 2021). Apart from its significant role in FA content modulation, there is little information on the biochemical characteristics of PDCT in major field crops including soybean. Herein, we identified two close homologues, namely, GmPDCT1 and GmPDCT2 of the reported AtPDCT (At3g15820) in the soybean genome (Figure 1a, Table S1). These homologues shared comparable gene composition and conserved motifs (Figure S1). Moreover, both homologues abundantly expressed proteins in the cytosol when ectopically expressed in the Arabidopsis mesophyll protoplasts (Figure 1b). In addition, the GmPDCT1 and GmPDCT2 genes were strongly upregulated in the mid-late stage developing seeds (Figure 1a, Table S2), which corroborated with the PDCTs expression profiles in other plants (Lu et al., 2009; Wickramarathna et al., 2015). To further elucidate the significance of GmPDCTs in the FAs composition of soybean seed oil, we generated two sgRNAs within the common GmPDCTs region, and generated CRISPR/Cas9 vectors for knock out experimentation (Figure 1c,d). Following the Agrobacterium tumefaciens-mediated transformation of soybean cultivar DN50, 15 positive T0 lines were acquired, among which, 7 (46.7%) simultaneously edited both GmPDCT1 and GmPDCT2 (Figure S2, Table S3). In all target sites, we observed single nucleotide insertion/deletion and relatively large deletions (7–128 bps) via PCR and Sanger sequencing (Figure 1e,f). In a majority of cases, the mutations produced premature translation termination, and this mutation was stably inherited in T1–T2 generations (Figures S2–S4). Lastly, no mutation events occurred across all potential off-target sites (Table S4). We selected three T2 homozygous mutants for additional investigations, and GmPDCT1 and GmPDCT2 transcripts in m3-1-1, m6-2-1 and m6-2-2 were strongly downregulated, relative to wildtype (WT) plants (Figure 1g). We employed western blot analysis using the PDCT polyclonal antibody to further confirm the GmPDCT protein knockout in mutant lines (Figure 1h). There were no discernible differences in seed size, seed weight or visible growth phenotypes of mutant, CK or WT lines (Figure S5, Table S5). In addition, the cotyledon cell structure, and total protein and oil contents in the mutant seeds remained unaltered, relative to WT (Figure 1i,j). However, the gmpdct1gmpdct2 mutants displayed marked elevations in MUFA content, along with a considerable decrease in PUFAs content. In mutant seeds, the mean C18:1 content was 2.49 folds that of WT, and the C18:2 and C18:3 contents were strongly diminished by 38.69% and 97.47%, respectively (Figure 1j, Table S6). To elucidate changes in lipid metabolism in the gmpdct1gmpdct2 double mutants, we further assessed the lipid profiles of developing seeds using electrospray ionization tandem mass spectrometry (ESI-MS/MS). Relative to WT, the MUFAs-containing TAGs were strongly elevated by 57.01% in the m3-1-1 and by 33.13% in the m6-2-1 mutants, respectively. In contrast, the TAG containing PUFAs C18:3 were strongly diminished, followed by C18:2 (Figure 1k, Table S7). Both DAG and PC exhibited a shift toward a more monounsaturated molecular species in the mutant lines (Figure 1k), as evidenced by the elevated 16:0/18:1 and 18:1/18:1 contents, with simultaneous decreases in the 16:0/18:2, 16:0/18:3, and 18:2/18:2 contents (Tables S8 and S9). It was previously reported that the TAG derived from PC-based DAG is laden with PUFAs, and the TAG derived from de novo DAG using glycerol-3-phosphate (G3P) acylation (Kennedy pathway) is rich in MUFAs (Eskandari et al., 2013). Herein, our TAG, DAG, and PC from GmPDCTs-deficient mutants revealed a steep increase in C18:1, along with a concomitant decrease in C18:2 and C18:3, suggesting that the de novo DAG network was upregulated while the PC-derived DAG was suppressed, resulting in very low incorporation of PC modified-PUFAs into TAG (Figure 1l). Based on these evidences, the PC to DAG turnover was potentially produced as a result of the catalytic action of PDCT in soybean developing seeds. In conclusion, herein, we successfully produced elevated-MUFAs soybean using GmPDCTs knockout via a CRISPR-Cas9 genome editing system. Our discovery of GmPDCTs provides numerous opportunities to modify plant oil composition for enhanced nutrition and shelf stability. This study was financially supported by NationalKey R&D Program of China (2021YFF1001202, 2021YFD1201104-02-02), NationalNatural Science Foundation of China (U20A2027, 31971899, 32272093, 32272072),and Natural Science Foundation of Heilongjiang Province (TD2022C003, JJ2022YX0475). The authors declare no conflicts of interest. H.L. and R.Z. performed most of the experiments and data analyses. P.L., M.Y., D.X., C.L. and Z.Z. provided technical support. X.W., Q.C. and Y.Z. wrote and revised the manuscript. All authors have read and approved the final manuscript. Figure S1 Identification and classification of PDCT gene family in soybean. Figure S2 DNA and deduced protein sequence of gene-edited mutants in T0 generation of GmPDCT1 and GmPDCT2 genes. Figure S3 DNA and deduced protein sequence of gene-edited mutants in T1 generation of GmPDCT1 and GmPDCT2 genes. Figure S4 DNA and deduced protein sequence of gene-edited mutants in T2 generation of GmPDCT1 and GmPDCT2 genes. Figure S5 Appearances comparison of seeds at different developmental stages between WT and T2 mutants. Table S1 Chromosomal locations and Phytozome gene IDs of PDCTs used in this study. Table S2 The expression of GmPDCTs in various tissues of wild type. Table S3 Editing events in 15 regenerated T0 plants, as determined by Sanger sequencing of plasmids derived from PCR products. Table S4 Analysis of potential off-target effects in edited mutants. Table S5 Comparison of agronomic traits of T3 mutants, CK and WT. Table S6 FA composition of mature seeds of the gmpdct1gmpdct2 mutants and WT soybean plants. Table S7 Molecular species of TAG from developing seeds at LM stage. Table S8 Molecular species of DAG from developing seeds at LM stage. Table S9 Molecular species of phospholipid from developing seeds at LM stage. Table S10 The primers used in this study. Please note: The publisher is not responsible for the content or functionality of any supporting information supplied by the authors. Any queries (other than missing content) should be directed to the corresponding author for the article.
更多查看译文
关键词
soybean (Glycine max (Linn,) Merr,),PDCT,CRISPR,Cas9,monounsaturated fatty acid,polyunsaturated fatty acid
AI 理解论文
溯源树
样例
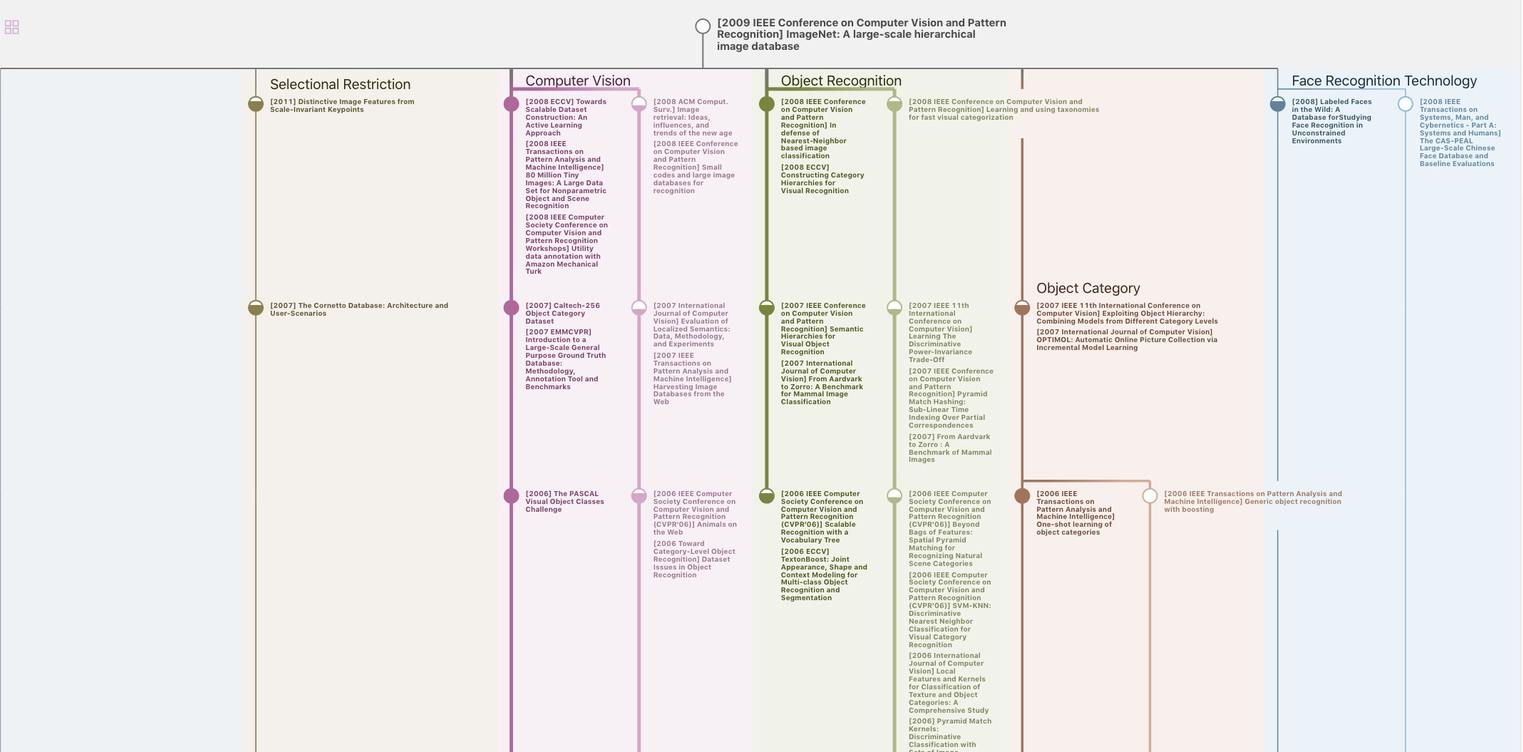
生成溯源树,研究论文发展脉络
Chat Paper
正在生成论文摘要