The Cardiovascular System in Cardiogenic Shock: Insight From a Cardiovascular Simulator
Pediatric critical care medicine : a journal of the Society of Critical Care Medicine and the World Federation of Pediatric Intensive and Critical Care Societies(2023)
摘要
THE CARDIOVASCULAR SYSTEM IN CARDIOGENIC SHOCK In the first article in this series on cardiovascular function during critical illness (1), we reviewed the ventricular pressure–volume relationship and time-varying elastance model and used a cardiovascular simulator to illustrate important principles of cardiovascular physiology and pathophysiology. We applied those principles to the assessment and management of septic shock. In this review, we take the same approach to illustrate important principles in the assessment and management of left ventricular (LV) systolic heart failure-induced cardiogenic shock. In common with sepsis, the hemodynamic profile of cardiogenic shock may vary considerably (2–4). Infection with the severe acute respiratory syndrome coronavirus 2 (SARS-CoV-2) exemplifies this point (5–9). In addition to causing hypoxic respiratory failure, SARS-CoV-2 can affect every aspect of the circulation. Direct viral injury of the myocardium and/or the inflammatory response to the virus may cause LV and/or right ventricular (RV) dysfunction. Vasomotor tone of the arterial resistance and venous capacitance vessels maybe altered as well. RV to pulmonary artery uncoupling may occur (8,9). Virus-induced endothelial injury and activation of the coagulation cascade resulting in a pulmonary embolism and/or positive pressure ventilation-induced increases in pulmonary vascular resistance may contribute to increases in RV afterload (8–10). Accordingly, the presentation may vary considerably, manifesting as cardiogenic shock or septic shock. Other lesions that may cause acute severe LV systolic heart failure include deterioration of chronic heart failure, valvular disease, and following cardiac surgery (so-called postcardiotomy cardiogenic shock). Importantly, the presentation of a patient depends on the chronicity of the lesion and the extent to which neurohormonal systems are activated (11). Regardless of the precipitating event, the common denominator is a limited stroke volume (SV) and cardiac output. There is no consensus on the optimal vasoactive regimen to treat systolic heart failure-induced cardiogenic shock in children or adults (3,4,12,13). The American Heart Association (AHA) 2022 statement on pediatric myocarditis recommends an inodilator such as milrinone as the first-line agent unless the patient is hypotensive in which case an inoconstrictor or vasopressor is recommended (12). The 2020 international consensus statement on cardiopulmonary resuscitation and emergency cardiovascular care in children published in Circulation makes the following recommendation: “It is reasonable to use epinephrine, levosimendan, dopamine or dobutamine for inotropic support in infants and children with cardiogenic shock (13).” The most recent AHA publications on adult myocarditis published in 2020 and adult cardiogenic shock published in 2017 emphasize the use of inoconstrictors such as norepinephrine and dopamine, and in some cases a vasopressor such as arginine vasopressin (AVP) or phenylephrine depending on the underlying cause and hemodynamic phenotype (3,4). With these broad recommendations in mind, we illustrate the impact of an inoconstrictor/vasoconstrictor strategy and contrast it with an inodilator/vasodilator strategy in the care of an adolescent patient in cardiogenic shock. Cardiogenic Shock Vignette A previously healthy 14-year-old male with a body weight of 50 kg and a body surface area of 1.5 m2 is admitted to the ICU with hypotension, tachypnea, and abdominal pain. The vital signs include a blood pressure (BP) 88 of 64 mm Hg, mean BP of 73 mm Hg, sinus tachycardia with a heart rate (HR) of 110 beats per minute, a pulse oximetry oxygen saturation (Spo2) of 94% on face-mask Fio2 of 0.4 and a temperature of 99oF. The physical examination is remarkable for a gallop, palpable pulses, warm extremities, and tachypnea. A chest radiograph shows cardiomegaly and diffuse interstitial pulmonary edema with areas of consolidation and an echocardiogram demonstrates severely depressed LV systolic function with an ejection fraction (EF) of 24% and minimally elevated LV end-diastolic volume (EDV). An indwelling arterial catheter and central venous line are placed. The central venous pressure (CVP) is 9 mm Hg, the central venous oxygen extraction ratio is 50%, the serum lactate level is normal, and the serum troponin level is markedly elevated. The initial diagnosis is cardiogenic shock due to myocarditis. Model Step 1: Baseline Hemodynamic State Figure 1 illustrates a hemodynamic profile consistent with acute myocarditis-induced cardiogenic shock with severely depressed LV contractility (LV end-systolic elastance, Ees, is significantly reduced). Systemic arterial elastance (Ea) is elevated due to neurohormonal activation and an increase in systemic vascular resistance (SVR) from 14 to 19 Wood Units [WU] and HR (60–110 bpm). The SV and EF are significantly decreased due to ventricular arterial uncoupling (Ea/Ees has risen from a normal value of 0.6 to nearly 3), the result of the increase in Ea and decrease in Ees (1). Due to the reduced EF and elevated end-systolic volume (ESV), venoconstriction, and an increase in the stressed blood volume (SBV), the EDV and end-diastolic pressure (EDP) (27 mm Hg) are elevated and responsible for hydrostatic pulmonary edema and impaired oxygenation (11,14,15). These findings contrast with the presentation of chronic systolic heart failure (Fig. 1), where ventricular remodeling results in a marked increase in EDV and a shift of the end diastolic pressure–volume relationship (EDPVR) down and to the right, indicative of a decrease in ventricular stiffness (increase in compliance). The LV pressure–volume loops (PVLs) illustrate that the EDP for each ventricle is similar despite disparate EDVs. Marked LV enlargement may also lead to functional mitral regurgitation and contribute importantly to the pathophysiology. In addition, LV contractility has decreased further and is indicated by the decrease in Ees and shift to the right of the volume axis intercept, Vo (1).Figure 1.: Ventricular pressure volume loops illustrating the development of acute severe left ventricular systolic heart failure, the effects of ventricular remodeling, and the development of chronic compensated left ventricular systolic heart failure. Ea = arterial elastance, EDP = end diastolic pressure, EDV = end diastolic volume, Ees = end systolic elastance, EDPVR = end diastolic pressure volume relationship, ESPVR = end systolic pressure volume relationship, ESV = end systolic volume, Pes = end systolic pressure, Vo = volume axis intercept.In this patient, ventricular stiffness has not changed from baseline (the EDPVR remains the same). The increase in LV EDP results from the increase in EDV and “operating stiffness” (1). Contributing to an increase in the LV filling pressure is impaired ventricular relaxation, which results from myocardial ischemia and impaired calcium resequestration by the sarcoplasm reticulum. Impaired relaxation is reflected in an upward shift of the diastolic pressure volume relationship without a change in slope (1). Incomplete relaxation would be reflected in the end diastolic pressure volume coordinate of the PVL not reaching the EDPVR before the onset of systole. The EDP of 27 mm Hg is 4 mm Hg greater than pulmonary artery occlusion pressure (PAOP), the latter measurement reflecting the mean left atrial pressure (16). The disparity between the EDP and PAOP increases as ventricular stiffness increases (16). The EDP and PAOP are disproportionately elevated relative to the CVP, demonstrating the lack of correlation between the right and left atrial pressures, and highlighting the limitation of inferring the LV filling pressure from a CVP (17). Additionally, despite the perfusion examination, the SVR is elevated at 19 WU, highlighting the potential discordance between estimations of and measured values of arterial peripheral resistance (18–20). Despite the severe hypotension and decrease in LV function, the serum lactate level is normal because the oxygen extraction ratio of 50% is less than the critical oxygen extraction ratio of 65% (21). Nonetheless, CO is significantly reduced (5.1 to 3.4 L/min). The mean BP exceeds the consensus-driven recommended minimum value of greater than 65 mm Hg for an adult (3,4,22). There is no consensus-driven recommendation for BP in children (23,24). The 5th to 50th percentile based on age is a mean BP of 60 to 70 mm Hg (23,24). With either approach, the coronary perfusion pressure (CPP; aortic diastolic BP—LV diastolic pressure) of 41 mm Hg is at the lower end of the consensus-driven recommended range of greater than 40–50 mm Hg (3,4,25) due to the elevated EDP. This finding demonstrates the importance of considering the downstream pressure for coronary perfusion (LV diastolic pressure or PAOP) when considering the adequacy of a CPP, and when selecting therapeutic interventions that affect not only the PAOP but also myocardial oxygen consumption (VO2) (e.g., volume expansion, vasoactive agents, mechanical circulatory support) (1). Model Step 2a: Inodilator Consider the theoretical response to an inodilator such as dobutamine, milrinone, or epinephrine (26–33). Figure 2A illustrates the theoretical impact of dobutamine such that Ees has increased by 15% and SVR has decreased from 19 to 16 WU (34,35). As a result of the decrease in Ea/Ees, the EF and SV have increased from the baseline state of cardiogenic shock (24–28%, 32–37 mL, respectively). The HR and CO have increased from 110 to 116 bpm and 3.5 to 4.2 L/min, respectively. Despite a decrease in SVR and Ea, the BP has increased due to a greater increase in Ees (mean BP and CPP have increased from 73 to 80 and 41 to 49 mm Hg, respectively). The increase in CPP is in part due to a decrease in the PAOP (23 to 21 mm Hg), the result of a decrease in the LV ESV, and vasodilation of the venous capacitance vessels, and decrease in SBV (11,14,15).Figure 2.: Ventricular pressure volume loops illustrating: the impact of dobutamine (DB) in acute left ventricular systolic heart failure followed by the addition of nitroprusside (NTP) (A); and the impact of dopamine (DA) in acute left ventricular systolic heart failure followed by the addition of arginine vasopressin (AVP) (B). Ea = arterial elastance, Ees = end-systolic elastance, EDPVR = end-diastolic pressure–volume relationship, Vo = volume axis intercept.Model Step 2b: Addition of a Vasodilator The addition of nitroprusside (NTP) an arterial-venous dilator is illustrated in Figure 2A. Additional vasodilation of arterial resistance and venous capacitance vessels, shifts the pressure PVL down and to the left, while further improving ventricular arterial coupling by decreasing Ea. The SV and CO have increased further (from 37 to 39 ml and 4.2 to 4.5 L/min), maintaining a mean BP of 75 mm Hg (36,37). Although the aortic diastolic pressure has decreased from 70 to 66 mm Hg, the CPP remains unchanged as the PAOP has decreased further. Meanwhile, LV myocardial VO2 has decreased as the pressure–volume area is reduced. Model Step 3a: Inoconstrictor Now consider the theoretical response to an inoconstrictor such as dopamine or norepinephrine. Figure 2B illustrates the impact of dopamine such that Ees increases to a similar extent as it did with the inodilator and SVR increases from 18 to 24 WU (34,35). The perfusion pressures have increased significantly: BP 88 of 64 mm Hg (with a mean of 73 mm Hg) to 104 of 84 mm Hg (with a mean of 92 mm Hg) and CPP from 41 to 55 mm Hg. Despite this change, and despite the increase in Ees, the EF and SV have not changed appreciably due to Ea increasing to a similar extent as Ees. CO remains unchanged. Due to an increase in ESV and venoconstriction and an increase in the SBV, the EDP, and PAOP have increased further (27–29 and 23–25 mm Hg, respectively) worsening lung injury and function (11,14,15,36,37). Model Step 3b: Addition of a Vasoconstrictor Figure 2B also illustrates the theoretical impact of adding a vasoconstrictor such as AVP to the inoconstrictor. An isolated increase in Ea further increases the extent of ventricular arterial uncoupling. As a result of the rise in Ea/Ees, the EF, SV, and CO decrease further (21 to 16%, 29 to 23 mL, and 3.5 to 2.8 L/min, respectively). Although the mean BP and CPP increase further and approach baseline normal values, so too have the LV EDV/EDP and PAOP (from 29 to 33 and 25 to 29 mm Hg, respectively), which is illustrated by a further shift in the PVL up and to the right along the EDPVR (11,14,15,36,37). When comparing the two strategies, EF, SV, and CO are much higher and the EDP much lower with the inodilator than inoconstrictor strategy: 31 versus 16%, 39 versus 23 mL, 4.5 versus 2.8 L/min, and 21 versus 33 mm Hg, respectively. Mechanical efficiency (stroke work [SW]/PVA) and LV myocardial VO2 are much more favorable with the inodilator strategy because Ea/Ees was considerably lower with each intervention relative to the inoconstrictor strategy (1). As the ventricle becomes uncoupled from its arterial system (i.e., Ea increases and/or Ees decreases), the proportion of the PVA that is wasted as potential energy increases and mechanical efficiency decreases (1). Furthermore, as Ea increases and SW is converted from volume work (SV) to pressure work, myocardial VO2 increases. The VO2 of the pressure-loaded contraction is considerably greater than that of the volume-loaded contraction performing comparable SW (1). Considering the similar changes in LV Ees and respective changes in Ea, the combination of dopamine and AVP generated an SW/PVA (2,346/7,860) of 30%, whereas the combination of dobutamine and nitroprusside generated an SW/PVA (3,120/6,182) of 50%. The net effect with similar Ees for each strategy is an LV myocardial VO2 that is lower with the inodilator versus inoconstrictor strategy. MECHANICAL CIRCULATORY SUPPORT Pharmacological therapies alone may not restore and maintain adequate myocardial and systemic oxygen delivery in which case an acute mechanical circulatory support (AMCS) device is indicated. The optimal selection of an AMCS device and the timing of its placement remains unknown and significant practice variability exists. These devices may be deployed as a bridge to recovery, decision, or long-term support devices. The approach to mechanical circulatory support should also consider optimizing myocardial protection and preservation while minimizing injury to the lung. The impact of AMCS devices on cardiovascular and respiratory systems will be discussed in a further PCCM Concise Clinical Physiology Review. CONCLUSIONS The ventricular pressure–volume relationship and time-varying elastance model and the application of a cardiovascular simulator enhance our understanding of disease processes; highlight the limitations of standard hemodynamic monitoring; illustrate the role of advanced hemodynamic monitoring; and enable us to predict the impact of therapies on clinical parameters used by the clinician at the bedside.
更多查看译文
关键词
arterial elastance,cardiogenic shock,cardiovascular physiology,pediatrics,ventricular elastance
AI 理解论文
溯源树
样例
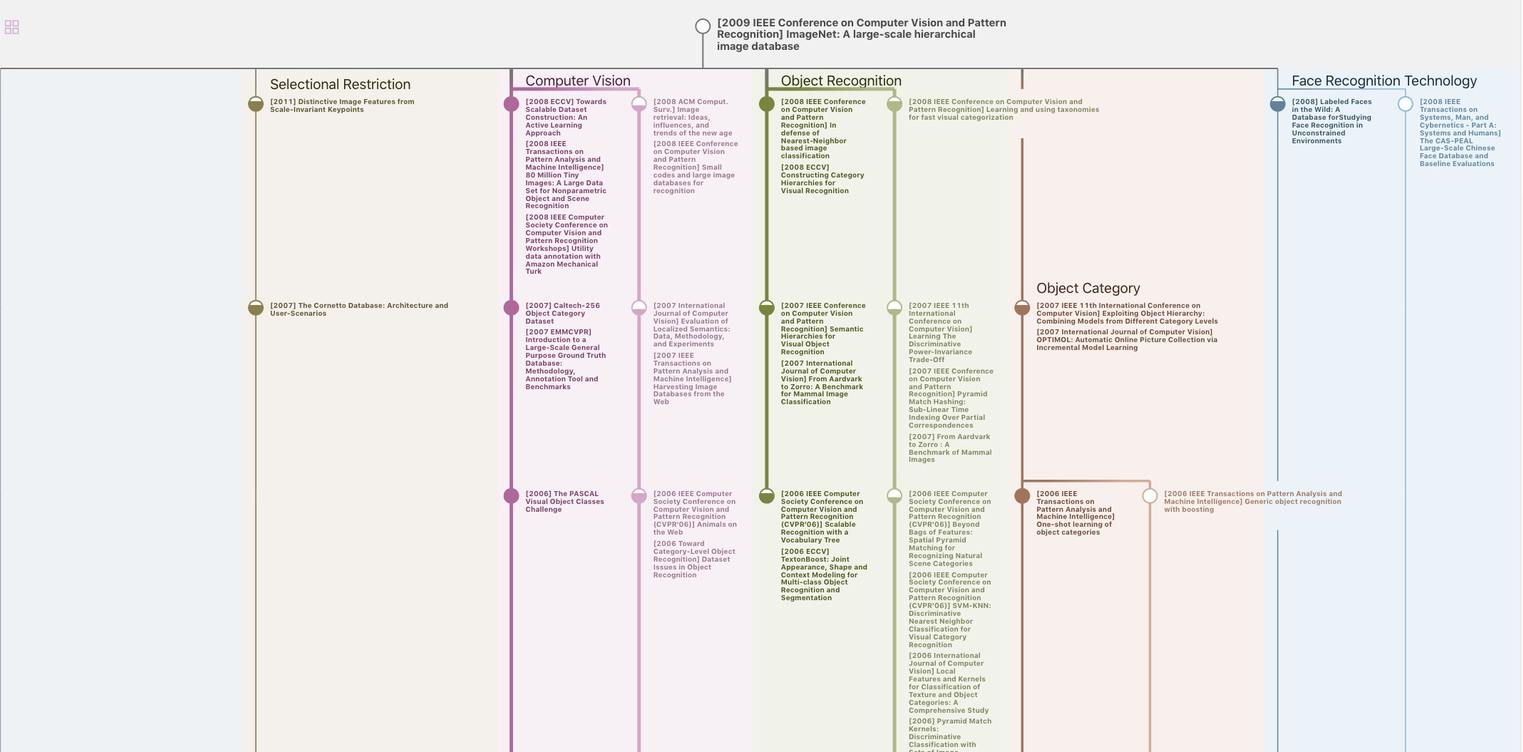
生成溯源树,研究论文发展脉络
Chat Paper
正在生成论文摘要