Chemical genetic identification of CDKL 5 substrates reveals its role in neuronal microtubule dynamics
The EMBO Journal(2018)
摘要
Article28 September 2018Open Access Source DataTransparent process Chemical genetic identification of CDKL5 substrates reveals its role in neuronal microtubule dynamics Lucas L Baltussen Lucas L Baltussen orcid.org/0000-0001-9742-6857 Kinases and Brain Development Laboratory, The Francis Crick Institute, London, UK Search for more papers by this author Priscilla D Negraes Priscilla D Negraes orcid.org/0000-0001-7876-1895 Department of Pediatrics, School of Medicine, University of California San Diego, La Jolla, CA, USA Search for more papers by this author Margaux Silvestre Margaux Silvestre orcid.org/0000-0003-1377-477X Kinases and Brain Development Laboratory, The Francis Crick Institute, London, UK Search for more papers by this author Suzanne Claxton Suzanne Claxton Kinases and Brain Development Laboratory, The Francis Crick Institute, London, UK Search for more papers by this author Max Moeskops Max Moeskops Kinases and Brain Development Laboratory, The Francis Crick Institute, London, UK Search for more papers by this author Evangelos Christodoulou Evangelos Christodoulou orcid.org/0000-0001-8464-1558 Structural Biology Science Technology Platform, The Francis Crick Institute, London, UK Search for more papers by this author Helen R Flynn Helen R Flynn orcid.org/0000-0001-7002-9130 Proteomics Science Technology Platform, The Francis Crick Institute, London, UK Search for more papers by this author Ambrosius P Snijders Ambrosius P Snijders Proteomics Science Technology Platform, The Francis Crick Institute, London, UK Search for more papers by this author Alysson R Muotri Corresponding Author Alysson R Muotri [email protected] orcid.org/0000-0003-0867-2875 Department of Pediatrics, School of Medicine, University of California San Diego, La Jolla, CA, USA Department of Pediatrics/Cellular & Molecular Medicine, Center for Academic Research and Training in Anthropogeny (CARTA), Kavli Institute for Brain and Mind, School of Medicine, Rady Children's Hospital San Diego, University of California San Diego, La Jolla, CA, USA Search for more papers by this author Sila K Ultanir Corresponding Author Sila K Ultanir [email protected] orcid.org/0000-0001-5745-3957 Kinases and Brain Development Laboratory, The Francis Crick Institute, London, UK Search for more papers by this author Lucas L Baltussen Lucas L Baltussen orcid.org/0000-0001-9742-6857 Kinases and Brain Development Laboratory, The Francis Crick Institute, London, UK Search for more papers by this author Priscilla D Negraes Priscilla D Negraes orcid.org/0000-0001-7876-1895 Department of Pediatrics, School of Medicine, University of California San Diego, La Jolla, CA, USA Search for more papers by this author Margaux Silvestre Margaux Silvestre orcid.org/0000-0003-1377-477X Kinases and Brain Development Laboratory, The Francis Crick Institute, London, UK Search for more papers by this author Suzanne Claxton Suzanne Claxton Kinases and Brain Development Laboratory, The Francis Crick Institute, London, UK Search for more papers by this author Max Moeskops Max Moeskops Kinases and Brain Development Laboratory, The Francis Crick Institute, London, UK Search for more papers by this author Evangelos Christodoulou Evangelos Christodoulou orcid.org/0000-0001-8464-1558 Structural Biology Science Technology Platform, The Francis Crick Institute, London, UK Search for more papers by this author Helen R Flynn Helen R Flynn orcid.org/0000-0001-7002-9130 Proteomics Science Technology Platform, The Francis Crick Institute, London, UK Search for more papers by this author Ambrosius P Snijders Ambrosius P Snijders Proteomics Science Technology Platform, The Francis Crick Institute, London, UK Search for more papers by this author Alysson R Muotri Corresponding Author Alysson R Muotri [email protected] orcid.org/0000-0003-0867-2875 Department of Pediatrics, School of Medicine, University of California San Diego, La Jolla, CA, USA Department of Pediatrics/Cellular & Molecular Medicine, Center for Academic Research and Training in Anthropogeny (CARTA), Kavli Institute for Brain and Mind, School of Medicine, Rady Children's Hospital San Diego, University of California San Diego, La Jolla, CA, USA Search for more papers by this author Sila K Ultanir Corresponding Author Sila K Ultanir [email protected] orcid.org/0000-0001-5745-3957 Kinases and Brain Development Laboratory, The Francis Crick Institute, London, UK Search for more papers by this author Author Information Lucas L Baltussen1,‡, Priscilla D Negraes2,‡, Margaux Silvestre1, Suzanne Claxton1, Max Moeskops1, Evangelos Christodoulou3, Helen R Flynn4, Ambrosius P Snijders4, Alysson R Muotri *,2,5 and Sila K Ultanir *,1 1Kinases and Brain Development Laboratory, The Francis Crick Institute, London, UK 2Department of Pediatrics, School of Medicine, University of California San Diego, La Jolla, CA, USA 3Structural Biology Science Technology Platform, The Francis Crick Institute, London, UK 4Proteomics Science Technology Platform, The Francis Crick Institute, London, UK 5Department of Pediatrics/Cellular & Molecular Medicine, Center for Academic Research and Training in Anthropogeny (CARTA), Kavli Institute for Brain and Mind, School of Medicine, Rady Children's Hospital San Diego, University of California San Diego, La Jolla, CA, USA ‡These authors contributed equally to this work *Corresponding author. Tel: +1 858 534 9320; E-mail: [email protected] *Corresponding author. Tel: +44 20 3796 1613; E-mail: [email protected] The EMBO Journal (2018)37:e99763https://doi.org/10.15252/embj.201899763 See also: IM Muñoz et al (December 2018) and PA Eyers (December 2018) PDFDownload PDF of article text and main figures. Peer ReviewDownload a summary of the editorial decision process including editorial decision letters, reviewer comments and author responses to feedback. ToolsAdd to favoritesDownload CitationsTrack CitationsPermissions ShareFacebookTwitterLinked InMendeleyWechatReddit Figures & Info Abstract Loss-of-function mutations in CDKL5 kinase cause severe neurodevelopmental delay and early-onset seizures. Identification of CDKL5 substrates is key to understanding its function. Using chemical genetics, we found that CDKL5 phosphorylates three microtubule-associated proteins: MAP1S, EB2 and ARHGEF2, and determined the phosphorylation sites. Substrate phosphorylations are greatly reduced in CDKL5 knockout mice, verifying these as physiological substrates. In CDKL5 knockout mouse neurons, dendritic microtubules have longer EB3-labelled plus-end growth duration and these altered dynamics are rescued by reduction of MAP1S levels through shRNA expression, indicating that CDKL5 regulates microtubule dynamics via phosphorylation of MAP1S. We show that phosphorylation by CDKL5 is required for MAP1S dissociation from microtubules. Additionally, anterograde cargo trafficking is compromised in CDKL5 knockout mouse dendrites. Finally, EB2 phosphorylation is reduced in patient-derived human neurons. Our results reveal a novel activity-dependent molecular pathway in dendritic microtubule regulation and suggest a pathological mechanism which may contribute to CDKL5 deficiency disorder. Synopsis Chemical genetic identification of physiological CDKL5 substrates EB2 and MAP1S reveals its role as a regulator of neuronal microtubule dynamics. MAP1S phosphorylation decreases microtubule affinity, suggesting a possible pathogenic pathway of CDKL5 deficiency disorder (CDD). CDKL5 directly phosphorylates the RPXS* motif of microtubule-associated proteins EB2, MAP1S and ARHGEF2. EB2 and MAP1S phosphorylation is lost in CDKL5 KO mouse brains and human CDD patient-derived neurons. MAP1S phosphorylation by CDKL5 directly impairs its microtubule binding and stabilizing properties. CDKL5 regulates dendritic microtubule dynamics in a MAP1S-dependent manner, resulting in altered cargo trafficking. Introduction Cyclin-dependent kinase-like 5 (CDKL5) is an X-linked gene encoding a 115 kDa serine/threonine kinase, a member of the CMGC (CDK, MAPK, GSK3 and CLK containing) kinase family (Manning et al, 2002). Mutations in a single copy of the CDKL5 gene cause a rare neurodevelopmental disorder predominantly observed in girls (Kalscheuer et al, 2003; Tao et al, 2004; Weaving et al, 2004; Scala et al, 2005). Although initially categorized as an atypical Rett syndrome, CDKL5 deficiency is now considered a distinct disorder, named CDKL5 deficiency disorder (CDD). CDD is characterized by early-onset seizures and widespread neurodevelopmental delay (Bahi-Buisson & Bienvenu, 2012; Fehr et al, 2013). Pathological deletions and nonsense mutations are found throughout the CDKL5 gene, whereas disease-causing missense variants are highly enriched in the N-terminal kinase domain of CDKL5, highlighting the importance of its kinase activity (Fehr et al, 2016). In mice, CDKL5 is expressed throughout the brain starting at late embryonic stages and through adulthood, indicating a role in postnatal development and function in the nervous system (Chen et al, 2010; Hector et al, 2016, 2017). CDKL5 mRNA is highly enriched in foetal and adult human brains (Hector et al, 2016). CDKL5's functions in excitatory and inhibitory synaptic transmission as well as dendrite, axon and spine development were studied using shRNA-mediated knockdown or CDKL5 knockout mice (Baltussen et al, 2017; Zhou et al, 2017). In addition, patient-derived induced pluripotent stem cells (hiPSCs) were used to obtain postmitotic neurons from patients with CDKL5 mutations (Amenduni et al, 2011; Ricciardi et al, 2012). However, no clear consensus has emerged on the cellular and molecular functions of CDKL5. Specifically, its direct physiological substrates are unknown. The microtubule cytoskeleton is required for fundamental cellular processes such as long-range intracellular transport and for dynamic cellular changes such as neural migration or neurite formation (Conde & Caceres, 2009; Kapitein & Hoogenraad, 2015). Microtubules in dendrites are oriented in mixed polarity (Baas et al, 1988). Microtubule plus-end growth visualized by fluorescently tagged end-binding proteins in living neurons helped characterize microtubule dynamics in dendrites (Stepanova et al, 2003; van de Willige et al, 2016; Yau et al, 2016). In primary neurons, dendritic neuronal activity and synaptic plasticity manipulations alter microtubule dynamics (Hu et al, 2008; Jaworski et al, 2009; Kapitein et al, 2011; Merriam et al, 2011; Ghiretti et al, 2016). Microtubule architecture and dynamics influence cargo trafficking on dendrites and axons (Yogev et al, 2016; Tas et al, 2017). Microtubules play central roles during neuronal development and function, and however, signalling pathways that regulate microtubule architecture, dynamics and microtubule-dependent trafficking remain to be elucidated. In this study, we identify CDKL5's direct substrates in mouse brain using a chemical genetic method (Hertz et al, 2010; Ultanir et al, 2012, 2014). We find that CDKL5 phosphorylates three microtubule-binding proteins, Rho guanine nucleotide exchange factor 2 (ARHGEF2), microtubule-associated protein 1S (MAP1S) and microtubule-associated protein RP/EB family member 2 (MAPRE2/EB2), implicating CDKL5 in microtubule-dependent processes. We show that EB2 Ser222 and MAP1S Ser812 phosphorylations are dramatically reduced in the CDKL5 knockout mouse brain, making them bona fide phosphorylation sites for CDKL5. EB2 phosphorylation is detected predominantly in dendrites of excitatory neurons. By imaging dendritic microtubule dynamics using EB3-tdTomato, we show that CDKL5 KO neurons have enhanced duration of plus-end growth, an effect that is dependent on MAP1S's direct binding to microtubules. We find specific defects in anterograde cargo trafficking of TrkB puncta. Interestingly, novel substrate phosphorylation is also highly reduced in patient iPSC-derived neurons, indicating that these substrates are conserved and are affected in human patients. Finally, we provide evidence that CDKL5-dependent phosphorylations are NMDAR activity dependent, suggesting a role in activity-dependent circuit formation. Our findings describe a novel regulatory mechanism on microtubules that is compromised in CDKL5 deficiency disorder. Results Chemical genetic identification of CDKL5 substrates MAP1S, EB2 and ARHGEF2 We used a chemical genetic approach to determine CDKL5's substrates in mouse brain lysates (Fig 1A; Blethrow et al, 2004; Hertz et al, 2010). This method utilizes analog-specific (AS) kinases, which can accept bulky ATP analogs owing to their enlarged ATP binding pocket. The gamma phosphate in bulky ATP analogs is replaced with a thiophosphate allowing the AS-kinase to thiophosphorylate its substrates. Bio-orthogonal bulky ATPγS analogs cannot be effectively used by wild-type kinases present in biological samples, allowing thiophosphorylation and labelling to be specific for the AS-kinase. First, to obtain an enlarged ATP binding pocket, we mutated the gatekeeper residue Phenylalanine 89 to a smaller amino acid Alanine (F89A) in CDKL5 kinase domain (amino acids 1–352; Appendix Fig S1A; Shah et al, 1997). Next, we tested the mutation's effect on CDKL5's autophosphorylation activity using a non-radioactive kinase assay utilizing ATPγS and its analog benzyl-ATPγS. Thiophosphate is reacted with p-nitro-benzyl-mesylate to obtain an epitope that is detected by a thiophosphate ester antibody (Allen et al, 2007). We found that F89A mutation enabled CDKL5 to utilize benzyl-ATPγS, albeit at low levels (Fig 1B and C). We engineered a second-site suppressor mutation C152A, which had been used to rescue NDR1 kinase (Ultanir et al, 2012; Appendix Fig S1B), and found that CDKL5 F89A/C152A activity with benzyl-ATPγS was comparable to wild-type kinase activity utilizing ATPγS (Fig 1B and C). CDKL51–352 F89A/C152A double mutant (from here onwards referred to as AS-CDKL5) could also thiophosphorylate Amphiphysin-1 (AMPH1), confirming its activity towards a known in vitro substrate (Sekiguchi et al, 2013; Appendix Fig S1C). We purified AS-CDKL5 either from HEK293 cells or from insect cells. Figure 1. Identification of CDKL5 substrates using chemical genetics A. Schematic of chemical genetic kinase substrate identification method. AS-CDKL5 utilizes benzyl-ATPγS to thiophosphorylate its substrates. Following trypsin digest, thiol-containing peptides are captured on iodoacetyl agarose beads. Thiophosphate ester linked peptides are released by oxone induced hydrolysis while cysteine-containing peptides remain attached. Eluted phosphopeptides are analysed with liquid chromatography-tandem mass spectrometry (LC-MS/MS). B, C. CDKL51–352 autophosphorylation is shown. WT CDKL5 uses ATPγS, KD is inactive, F89A uses benzyl-ATPγS with reduced efficiency, F89A/C152A mutant has rescued activity. Quantification of Thio-P signal is normalized for CDKL5 levels (HA), and KD background is subtracted. Fisher's LSD: n = 4 replicates. bn, benzyl. D. A list of novel CDKL5 substrate targets and phosphorylation sites identified. All phosphorylation sites are conserved in human. # MAP1S pS812 is identified based on sequence homology. Red = phosphorylated serine, bold = RPXS consensus sequence. E–H. In vitro kinase assays showing efficient MAP1S phosphorylation by CDKL5. 50 ng (40 nM) AS-CDKL5 phosphorylates 150 ng (50 nM) MAP1S very rapidly (E, F). In 30 min of incubation, 150 ng MAP1S is phosphorylated by small amounts of CDKL5 (G, H). Quantification of phosphorylated MAP1S is normalized to maximum intensity. n = 2 replicates. I–L. Western blots of in vitro kinase assays showing loss of phosphorylation in phosphomutants ARHGEF2 S122A (I), EB2 S222A (J) and MAP1S S786/812A (K). Substrate levels are shown by Coomassie staining underneath. MAP1S phosphorylation is quantified in (L). Dunnett's multiple comparison: n = 3 replicates. Data information: Thio-P, anti-thiophosphate ester antibody; n.s., not significant, *P < 0.05, ***P < 0.001, ****P < 0.0001, error bars are SEM. In panel (K), non-relevant lanes have been removed for simplicity (see accompanying Source Data for full blot). Source data are available online for this figure. Source Data for Figure 1 [embj201899763-sup-0009-SDataFig1.pdf] Download figure Download PowerPoint In order to label CDKL5 substrates, we reacted purified AS-CDKL5 and benzyl-ATPγS with 1–2 mg of postnatal day 12 (P12) mouse brain lysate. Thiophosphorylated substrate proteins were digested to peptide fragments, and thiophosphopeptides were purified and identified using mass spectrometry (Fig 1A). We used kinase-dead (KD) CDKL5 (K42A) as negative control and conducted three independent experiments totalling eight AS replicates. We repeatedly detected ARHGEF2 Ser122, EB2 Ser222 and MAP1S Ser786 phosphopeptides specifically in AS-kinase samples and not in negative controls (Fig EV1, Appendix Figs S2 and S3). All phosphorylation sites contained an RPXS* consensus motif, where X is any amino acid and S* is the phosphorylated residue (Fig 1D). To validate these putative substrates, we performed in vitro kinase assays using purified AS-CDKL5 and purified substrates. We found that all three substrates are highly efficiently phosphorylated by CDKL5 in a time-dependent (Fig 1E and F, and Appendix Fig S4) and CDKL5 concentration-dependent manner (Fig 1G and H, and Appendix Fig S4). Next, to confirm the identified phosphorylation sites, we generated phosphomutant substrates by mutating the Serines to Alanines and performed in vitro kinase assays. We found that ARHGEF2 S122A (Fig 1I) and EB2 S222A (Fig 1J) are not phosphorylated by CDKL5, confirming the mutated Serines as the sole phosphorylation sites on these substrates. In contrast, full-length MAP1S phosphorylation was reduced in MAP1S S786A mutant but not completely eliminated. Upon inspection of MAP1S protein sequence, we determined another putative phosphorylation site with the same RPXS consensus motif at Ser812. MAP1S phosphorylation was lost in double phosphomutant S786/812A, indicating that CDKL5 can phosphorylate MAP1S on both sites (Fig 1K and L). In summary, our chemical genetic screen identified three novel CDKL5 substrates on which four phosphorylation sites containing RPXS* motif were confirmed (Fig 1D). Interestingly, all substrates are microtubule-associated proteins, suggesting a role for CDKL5 in microtubule regulation. Click here to expand this figure. Figure EV1. MS2 spectra of CDKL5 substrates A–C. Best identification spectra for ARHGEF2 (A), EB2 (B) and MAP1S (C) isolated by chemical genetic covalent capture for CDKL5 substrates. Sequences are determined by product ions of b- and y-series after collision-induced dissociation (CID) or higher-energy C-trap dissociation (HCD). Neutral loss of phosphoric acid generates a product ion series that corresponds only to those ions containing the phosphorylated residue (*). # of repeats represent the number of CDKL5 AS samples in which the specific phosphosite was identified. Only phosphosites never identified in KD samples were considered putative CDKL5 targets. Download figure Download PowerPoint MAP1S and EB2 are physiological substrates of CDKL5 in brain In order to study the phosphorylation events more closely, we generated rabbit polyclonal phosphospecific antibodies targeting the four mouse phosphorylation sites that we identified (Fig 1D). We expressed wild-type (WT) and phosphomutant substrates, ARHGEF2, EB2 and MAP1S, together with full-length wild-type or kinase-dead CDKL5 in HEK293 cells. We found that ARHGEF2 Ser122 and MAP1S Ser786 phosphorylation levels were increased with CDKL5 overexpression, demonstrating that these substrates can be phosphorylated by CDKL5 in cells, while EB2 Ser222 and MAP1S Ser812 sites were already highly phosphorylated endogenously (Fig EV2A–C). Importantly, for all four cases, phosphospecific antibodies recognized the WT but not the phosphomutant substrates, indicating their specificity for phosphorylated substrates. Click here to expand this figure. Figure EV2. Validation of phosphospecific antibodies A–C. HEK293 cells co-transfected with full-length WT or KD FLAG-CDKL5 and Strep-ARHGEF2 (A), HA-EB2 (B) or HA-MAP1S (C) are probed with their respective phosphospecific antibodies. Phosphospecific antibodies do not detect phosphomutants, indicating their specificity. ARHGEF2 pS122 (A) and MAP1S pS786 (C) are increased when WT CDKL5 is expressed. High levels of endogenous EB2 pS222 (B) and MAP1S pS812 (C) are not altered with WT CDKL5. Total levels of kinase (FLAG) and substrate (Strep/HA) are detected by epitope tags. D, E. Full molecular weight range of Western blots with mouse P20 cortical lysates probed for total EB2 and EB2 pS222 (D) and MAP1S light chain and MAP1S pS812 (E). F. Efficient shRNA-mediated knockdown of EB2 in rat primary neurons is shown by the specific loss of EB2 staining in transfected cells. Scrambled shRNA was used as a control. EB2 pS222 signal is apparent in dendrites of the control (arrowheads). The remaining nuclear signal of EB2 pS222 after shRNA-mediated knockdown (*) is considered non-specific. Scale bar is 10 μm. Source data are available online for this figure. Download figure Download PowerPoint Using these antibodies, we tested if the novel substrates are phosphorylated by CDKL5 in vivo in mice. CDKL5 protein was shown to be expressed in the cortex of wild-type (WT) mice and completely lost in CDKL5 knockout (KO) mice (Fig 2A). We found that endogenous EB2 Ser222 (pS222) and MAP1S Ser812 (pS812) phosphorylations are dramatically reduced in CDKL5 knockout mice cortex at P20 (Fig 2A and B). ARHGEF2 Ser122 and MAP1S Ser786 phosphospecific antibodies did not detect any specific signal, possibly due to insufficient sensitivity of these antibodies. These data indicated MAP1S and EB2 to be physiological substrates of CDKL5. Figure 2. MAP1S and EB2 are physiological substrates of CDKL5 in mammalian brain A, B. In vivo substrate phosphorylations are assessed by Western blotting of 5 WT (CDKL5+/Y) and 5 CDKL5 KO (CDKL5−/Y) mouse cortical lysates using phosphospecific antibodies. EB2 pS222 and MAP1S pS812 are dramatically reduced in KOs. Quantification of substrate phosphorylation is normalized for total protein level. Student's t-test: n = 5 animals per genotype. C, D. Developmental expression of CDKL5 and levels of its substrate phosphorylations in P4–P50 cortex. CDKL5-dependent substrate phosphorylation is quantified by subtracting KO from WT levels for each age. n = 3 animals per age per genotype. E, F. Expression of CDKL5 and levels of substrate phosphorylation in P8 Nex-Cre conditional KO cortex. CDKL5 protein expression is almost completely lost. Quantification of substrate phosphorylation is normalized for total protein level. Student's t-test: n = 3 animals per genotype. G. CDKL5 WT and CDKL5 KO neurons in culture are co-stained with neuronal dendrite marker MAP2, EB2 and EB2 pS222. EB2 pS222 is lost in CDKL5 KO dendrites. Scale bar is 20 μm. Data information: EB2 isoform 1/2 (37 kD) is quantified. ****P < 0.0001, error bars are SEM. Source data are available online for this figure. Source Data for Figure 2 [embj201899763-sup-0010-SDataFig2.pdf] Download figure Download PowerPoint We also investigated the temporal and spatial distribution of CDKL5 activity. For this purpose, we examined the developmental time course of CDKL5 expression and of EB2 and MAP1S phosphorylation in mouse cortex. When comparing WT and KO cortical lysates from P4, P12, P20 and P50, we found that at all ages EB2 pS222 and MAP1S pS812 are highly dependent on CDKL5, as shown by absence of phosphorylations in CDKL5 KO (Figs 2C and D, and EV2D and E for full molecular range). Interestingly, while CDKL5 protein level increased postnatally and remained at its highest level in adult, CDKL5 activity towards EB2 pS222 and MAP1S pS812 was reduced significantly through 2nd to 3rd postnatal weeks. In order to assay phosphatase regulation of these substrates, we treated cultured neurons at younger (DIV11) or older (DIV22) developmental stages with PP1/PP2A type phosphatase inhibitor, okadaic acid (OA). We found that OA increased EB2 pS222 levels in both stages (Fig EV3A). The fold increase in EB2 pS222 was larger at DIV22 when compared to DIV11, indicating that PP1/PP2A activity may be higher in older stages than in younger neurons (Fig EV3B). However, even in the presence of OA the observed decrease in EB2 phosphorylation in older neurons remained, indicating that additional factors likely contribute to observed reduction in EB2 pS222 (Fig EV3C). Click here to expand this figure. Figure EV3. Changes in EB2 phosphorylation are not due to phosphatases or osmolarity A–C. Phosphatase activity does not attribute to the reduced EB2 phosphorylation in mature neurons. EB2 phosphorylation is reduced in mature rat primary cortical neurons at DIV22 compared to developing neurons at DIV11. Thirty-minute treatment with 1 μM okadaic acid (OA) inhibits phosphatases and increases EB2 pS222 signal. The fold change in EB2 pS222 after OA at DIV22 is trending to be larger than at DIV11 (B), but reduced EB2 phosphorylation at DIV22 is not completely rescued by phosphatase inhibition (C). Quantification of EB2 phosphorylation is normalized for total protein level. Student's t-test (B), Tukey's multiple comparison (C): n = 3 repeats, *P < 0.05, ***P < 0.001, error bars are SEM. D. NaCl treatment is a control for the changes in osmolarity induced by KCl without the depolarizing effect. Thirty-minute treatment of rat primary cortical neurons with 50 mM NaCl did not lead to the reduction in EB2 S222 phosphorylation observed with the same amount of KCl. Source data are available online for this figure. Download figure Download PowerPoint Next, we examined the cell-type specificity of CDKL5 activity by deleting CDKL5 in excitatory neurons using Nex-Cre mice (Goebbels et al, 2006) crossed with floxed CDKL5 mouse (Amendola et al, 2014). We observed that the vast majority of CDKL5 is found in excitatory neurons and that EB2 Ser222 and MAP1S Ser812 phosphorylations are significantly reduced at P8 in cortex of excitatory neuron-specific CDKL5 conditional knockouts (Fig 2E and F). In order to determine the subcellular distribution of phosphorylated CDKL5 substrates, we immunostained dissociated cortical neuron cultures with EB2 pS222. We found that total EB2 and EB2 pS222 were largely present in dendrites and EB2 pS222 staining was absent in CDKL5 KO cultures confirming its specificity (Fig 2G). In order to assess the specificity of EB2 pS222 staining, we knocked down endogenous EB2 using a previously reported shRNA sequence (Komarova et al, 2005). In contrast to scrambled shRNA control, EB2 shRNA caused a dramatic reduction in total EB2 and pS222 in dendrites of neurons expressing these plasmids along with GFP (Fig EV2F). Nuclear staining present with pS222 was non-specific background, as it was observed with total EB2 staining and it was not reduced by EB2 shRNA. These findings indicated that CDKL5 phosphorylates EB2 and MAP1S in vivo during early postnatal development and that CDKL5 is highly active in dendrites of pyramidal neurons in the cortex. EB2 Ser222 phosphorylation is suppressed by NMDA receptor activity Neuronal activity and NMDA receptors are crucial in sculpting developing neuronal circuits and for activity-dependent plasticity in adults (Wong & Ghosh, 2002). We next tested if CDKL5 substrate phosphorylation was affected by NMDAR-dependent neuronal activity. We treated cortical neuronal cultures with 50 mM KCl for varying durations to induce neuronal depolarization. We found that phosphorylation of EB2 Ser222 was reduced to 50% of its initial levels within 10 min of KCl treatment (Fig 3A and B). This effect was due to depolarization and not because of altered osmolarity (Appendix Fig S3C). Total CDKL5 levels were also reduced by 25% (Fig 3C). To test the role of NMDAR on EB2 pS222 regulation, we inhibited NMDAR with 100 μM AP5 during KCl treatment and found that AP5 largely rescued the KCl-mediated EB2 pS222 reduction (Fig 3A and B). Finally, we applied NMDA on cultured neurons and found that NMDA application reduced EB2 phosphorylation, supporting NMDAR-dependent regulation of CDKL5 substrate phosphorylation (Fig 3D and E). These data showed that CDKL5's phosphorylation of EB2 is inhibited by NMDAR activity, suggesting that CDKL5 contributes to activity-dependent circuit formation. Figure 3. Phosphorylation of EB2 Ser222 is suppressed by NMDAR activity A–C. Protein lysates were obtained from cortical neuronal cultures treated with 50 mM KCl applied directly to culture media for indicated durations. 100 μm AP5 was used to block NMDAR activity during KCl treatment. Quantification of phosphorylated EB2 (B) and total CDKL5 (C) is normalized to 0 min time point. EB2 phosphorylation is significantly decreased after 10 min of KCl treatment compared to 0 min. CDKL5 protein levels are slightly reduced after 40 min compared to 0 min. Dunnett's multiple comparison: n = 3 replicates. D, E. Protein lysates were obtained from cortical neuronal cultures treated with 50 μM NMDA applied directly to culture media for indicated durations. Quantification of phosphorylated EB2 and total CDKL5 is normalized to 0 min time point. EB2 S222 phosphorylation is significantly reduced after 10 min compared to 0 min. Dunnett's multiple comparison: n = 3 replicates. Data information: *P < 0.05, **P < 0.01, ***P < 0.001, error bars are SEM. Source d
更多查看译文
关键词
chemical genetic identification
AI 理解论文
溯源树
样例
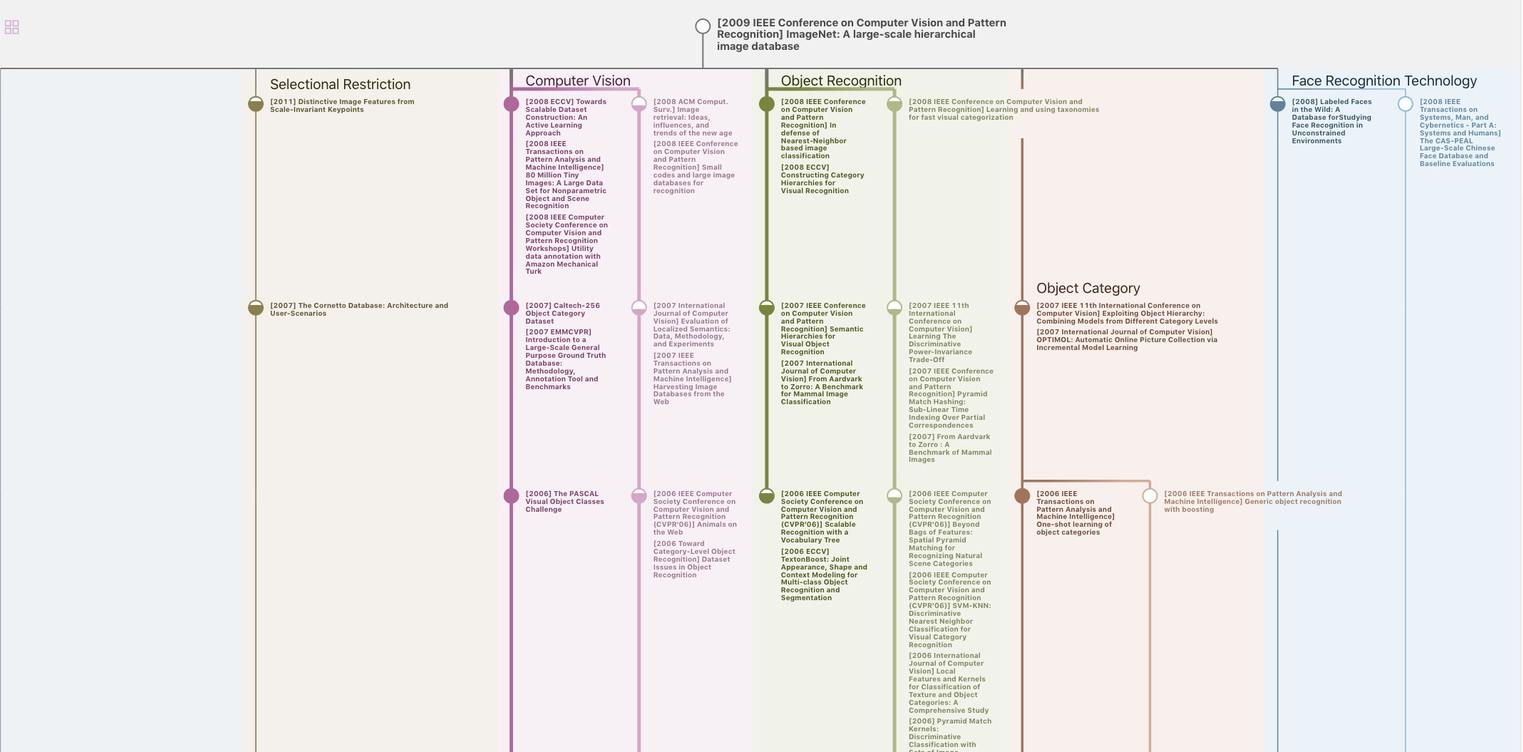
生成溯源树,研究论文发展脉络
Chat Paper
正在生成论文摘要