RNA editing of Filamin A pre‐ mRNA regulates vascular contraction and diastolic blood pressure
The EMBO Journal(2018)
摘要
Article7 August 2018Open Access Source DataTransparent process RNA editing of Filamin A pre-mRNA regulates vascular contraction and diastolic blood pressure Mamta Jain Corresponding Author Mamta Jain [email protected] Division of Cell Biology, Center for Anatomy and Cell Biology, Medical University of Vienna, Vienna, Austria Search for more papers by this author Tomer D Mann Tomer D Mann The Mina and Everard Goodman Faculty of Life Sciences, Bar Ilan University, Ramat-Gan, Israel Tel Aviv Sourasky Medical Center, Tel Aviv, Israel Search for more papers by this author Maja Stulić Maja Stulić Division of Cell Biology, Center for Anatomy and Cell Biology, Medical University of Vienna, Vienna, Austria Search for more papers by this author Shailaja P Rao Shailaja P Rao Center of Molecular Medicine, Institute of Molecular Biology and Biochemistry, Medical University of Graz, Graz, Austria Search for more papers by this author Andrijana Kirsch Andrijana Kirsch Center of Molecular Medicine, Institute of Molecular Biology and Biochemistry, Medical University of Graz, Graz, Austria Search for more papers by this author Dieter Pullirsch Dieter Pullirsch Division of Cell Biology, Center for Anatomy and Cell Biology, Medical University of Vienna, Vienna, Austria Search for more papers by this author Xué Strobl Xué Strobl Division of Cell Biology, Center for Anatomy and Cell Biology, Medical University of Vienna, Vienna, Austria Search for more papers by this author Claus Rath Claus Rath Division of Anatomy, Center for Anatomy and Cell Biology, Medical University of Vienna, Vienna, Austria Search for more papers by this author Lukas Reissig Lukas Reissig Division of Anatomy, Center for Anatomy and Cell Biology, Medical University of Vienna, Vienna, Austria Search for more papers by this author Kristin Moreth Kristin Moreth German Mouse Clinic, Institute of Experimental Genetics, Helmholtz Zentrum München, Neuherberg, Germany Search for more papers by this author Tanja Klein-Rodewald Tanja Klein-Rodewald German Mouse Clinic, Institute of Experimental Genetics, Helmholtz Zentrum München, Neuherberg, Germany Institute of Pathology, Helmholtz Zentrum München, Neuherberg, Germany Search for more papers by this author Raffi Bekeredjian Raffi Bekeredjian Department of Cardiology, University of Heidelberg, Heidelberg, Germany Search for more papers by this author Valerie Gailus-Durner Valerie Gailus-Durner German Mouse Clinic, Institute of Experimental Genetics, Helmholtz Zentrum München, Neuherberg, Germany Search for more papers by this author Helmut Fuchs Helmut Fuchs German Mouse Clinic, Institute of Experimental Genetics, Helmholtz Zentrum München, Neuherberg, Germany Search for more papers by this author Martin Hrabě de Angelis Martin Hrabě de Angelis German Mouse Clinic, Institute of Experimental Genetics, Helmholtz Zentrum München, Neuherberg, Germany Department of Experimental Genetics, Center of Life and Food Sciences Weihenstephan, Technische Universität München, Freising-Weihenstephan, Germany German Center for Diabetes Research (DZD), Neuherberg, Germany Search for more papers by this author Eleonore Pablik Eleonore Pablik Section for Medical Statistics, CeMSIIS, Medical University of Vienna, Vienna, Austria Search for more papers by this author Laura Cimatti Laura Cimatti Division of Cell Biology, Center for Anatomy and Cell Biology, Medical University of Vienna, Vienna, Austria Search for more papers by this author David Martin David Martin Division of Cell Biology, Center for Anatomy and Cell Biology, Medical University of Vienna, Vienna, Austria Search for more papers by this author Jelena Zinnanti Jelena Zinnanti Vienna Biocenter Core Facilities GmbH, Vienna, Austria Search for more papers by this author Wolfgang F Graier Wolfgang F Graier Center of Molecular Medicine, Institute of Molecular Biology and Biochemistry, Medical University of Graz, Graz, Austria Search for more papers by this author Maria Sibilia Maria Sibilia Department of Medicine I, Comprehensive Cancer Center, Institute for Cancer Research, Medical University of Vienna, Vienna, Austria Search for more papers by this author Saša Frank Saša Frank Center of Molecular Medicine, Institute of Molecular Biology and Biochemistry, Medical University of Graz, Graz, Austria Search for more papers by this author Erez Y Levanon Erez Y Levanon orcid.org/0000-0002-3641-4198 The Mina and Everard Goodman Faculty of Life Sciences, Bar Ilan University, Ramat-Gan, Israel Search for more papers by this author Michael F Jantsch Corresponding Author Michael F Jantsch [email protected] orcid.org/0000-0003-1747-0853 Division of Cell Biology, Center for Anatomy and Cell Biology, Medical University of Vienna, Vienna, Austria Search for more papers by this author Mamta Jain Corresponding Author Mamta Jain [email protected] Division of Cell Biology, Center for Anatomy and Cell Biology, Medical University of Vienna, Vienna, Austria Search for more papers by this author Tomer D Mann Tomer D Mann The Mina and Everard Goodman Faculty of Life Sciences, Bar Ilan University, Ramat-Gan, Israel Tel Aviv Sourasky Medical Center, Tel Aviv, Israel Search for more papers by this author Maja Stulić Maja Stulić Division of Cell Biology, Center for Anatomy and Cell Biology, Medical University of Vienna, Vienna, Austria Search for more papers by this author Shailaja P Rao Shailaja P Rao Center of Molecular Medicine, Institute of Molecular Biology and Biochemistry, Medical University of Graz, Graz, Austria Search for more papers by this author Andrijana Kirsch Andrijana Kirsch Center of Molecular Medicine, Institute of Molecular Biology and Biochemistry, Medical University of Graz, Graz, Austria Search for more papers by this author Dieter Pullirsch Dieter Pullirsch Division of Cell Biology, Center for Anatomy and Cell Biology, Medical University of Vienna, Vienna, Austria Search for more papers by this author Xué Strobl Xué Strobl Division of Cell Biology, Center for Anatomy and Cell Biology, Medical University of Vienna, Vienna, Austria Search for more papers by this author Claus Rath Claus Rath Division of Anatomy, Center for Anatomy and Cell Biology, Medical University of Vienna, Vienna, Austria Search for more papers by this author Lukas Reissig Lukas Reissig Division of Anatomy, Center for Anatomy and Cell Biology, Medical University of Vienna, Vienna, Austria Search for more papers by this author Kristin Moreth Kristin Moreth German Mouse Clinic, Institute of Experimental Genetics, Helmholtz Zentrum München, Neuherberg, Germany Search for more papers by this author Tanja Klein-Rodewald Tanja Klein-Rodewald German Mouse Clinic, Institute of Experimental Genetics, Helmholtz Zentrum München, Neuherberg, Germany Institute of Pathology, Helmholtz Zentrum München, Neuherberg, Germany Search for more papers by this author Raffi Bekeredjian Raffi Bekeredjian Department of Cardiology, University of Heidelberg, Heidelberg, Germany Search for more papers by this author Valerie Gailus-Durner Valerie Gailus-Durner German Mouse Clinic, Institute of Experimental Genetics, Helmholtz Zentrum München, Neuherberg, Germany Search for more papers by this author Helmut Fuchs Helmut Fuchs German Mouse Clinic, Institute of Experimental Genetics, Helmholtz Zentrum München, Neuherberg, Germany Search for more papers by this author Martin Hrabě de Angelis Martin Hrabě de Angelis German Mouse Clinic, Institute of Experimental Genetics, Helmholtz Zentrum München, Neuherberg, Germany Department of Experimental Genetics, Center of Life and Food Sciences Weihenstephan, Technische Universität München, Freising-Weihenstephan, Germany German Center for Diabetes Research (DZD), Neuherberg, Germany Search for more papers by this author Eleonore Pablik Eleonore Pablik Section for Medical Statistics, CeMSIIS, Medical University of Vienna, Vienna, Austria Search for more papers by this author Laura Cimatti Laura Cimatti Division of Cell Biology, Center for Anatomy and Cell Biology, Medical University of Vienna, Vienna, Austria Search for more papers by this author David Martin David Martin Division of Cell Biology, Center for Anatomy and Cell Biology, Medical University of Vienna, Vienna, Austria Search for more papers by this author Jelena Zinnanti Jelena Zinnanti Vienna Biocenter Core Facilities GmbH, Vienna, Austria Search for more papers by this author Wolfgang F Graier Wolfgang F Graier Center of Molecular Medicine, Institute of Molecular Biology and Biochemistry, Medical University of Graz, Graz, Austria Search for more papers by this author Maria Sibilia Maria Sibilia Department of Medicine I, Comprehensive Cancer Center, Institute for Cancer Research, Medical University of Vienna, Vienna, Austria Search for more papers by this author Saša Frank Saša Frank Center of Molecular Medicine, Institute of Molecular Biology and Biochemistry, Medical University of Graz, Graz, Austria Search for more papers by this author Erez Y Levanon Erez Y Levanon orcid.org/0000-0002-3641-4198 The Mina and Everard Goodman Faculty of Life Sciences, Bar Ilan University, Ramat-Gan, Israel Search for more papers by this author Michael F Jantsch Corresponding Author Michael F Jantsch [email protected] orcid.org/0000-0003-1747-0853 Division of Cell Biology, Center for Anatomy and Cell Biology, Medical University of Vienna, Vienna, Austria Search for more papers by this author Author Information Mamta Jain *,1,‡, Tomer D Mann2,3,‡, Maja Stulić1,‡, Shailaja P Rao4, Andrijana Kirsch4, Dieter Pullirsch1, Xué Strobl1, Claus Rath5, Lukas Reissig5, Kristin Moreth6, Tanja Klein-Rodewald6,7, Raffi Bekeredjian8, Valerie Gailus-Durner6, Helmut Fuchs6, Martin Hrabě de Angelis6,9,10, Eleonore Pablik11, Laura Cimatti1, David Martin1, Jelena Zinnanti12, Wolfgang F Graier4, Maria Sibilia13, Saša Frank4, Erez Y Levanon2 and Michael F Jantsch *,1 1Division of Cell Biology, Center for Anatomy and Cell Biology, Medical University of Vienna, Vienna, Austria 2The Mina and Everard Goodman Faculty of Life Sciences, Bar Ilan University, Ramat-Gan, Israel 3Tel Aviv Sourasky Medical Center, Tel Aviv, Israel 4Center of Molecular Medicine, Institute of Molecular Biology and Biochemistry, Medical University of Graz, Graz, Austria 5Division of Anatomy, Center for Anatomy and Cell Biology, Medical University of Vienna, Vienna, Austria 6German Mouse Clinic, Institute of Experimental Genetics, Helmholtz Zentrum München, Neuherberg, Germany 7Institute of Pathology, Helmholtz Zentrum München, Neuherberg, Germany 8Department of Cardiology, University of Heidelberg, Heidelberg, Germany 9Department of Experimental Genetics, Center of Life and Food Sciences Weihenstephan, Technische Universität München, Freising-Weihenstephan, Germany 10German Center for Diabetes Research (DZD), Neuherberg, Germany 11Section for Medical Statistics, CeMSIIS, Medical University of Vienna, Vienna, Austria 12Vienna Biocenter Core Facilities GmbH, Vienna, Austria 13Department of Medicine I, Comprehensive Cancer Center, Institute for Cancer Research, Medical University of Vienna, Vienna, Austria ‡These authors contributed equally to this work *Corresponding author. Tel: +43 1 40160 37752; E-mail: [email protected] *Corresponding author. Tel: +43 1 40160 37510; Fax: +43 1 40160 37542; E-mail: [email protected] The EMBO Journal (2018)37:e94813https://doi.org/10.15252/embj.201694813 PDFDownload PDF of article text and main figures. Peer ReviewDownload a summary of the editorial decision process including editorial decision letters, reviewer comments and author responses to feedback. ToolsAdd to favoritesDownload CitationsTrack CitationsPermissions ShareFacebookTwitterLinked InMendeleyWechatReddit Figures & Info Abstract Epitranscriptomic events such as adenosine-to-inosine (A-to-I) RNA editing by ADAR can recode mRNAs to translate novel proteins. Editing of the mRNA that encodes actin crosslinking protein Filamin A (FLNA) mediates a Q-to-R transition in the interactive C-terminal region. While FLNA editing is conserved among vertebrates, its physiological function remains unclear. Here, we show that cardiovascular tissues in humans and mice show massive editing and that FLNA RNA is the most prominent substrate. Patient-derived RNA-Seq data demonstrate a significant drop in FLNA editing associated with cardiovascular diseases. Using mice with only impaired FLNA editing, we observed increased vascular contraction and diastolic hypertension accompanied by increased myosin light chain phosphorylation, arterial remodeling, and left ventricular wall thickening, which eventually causes cardiac remodeling and reduced systolic output. These results demonstrate a causal relationship between RNA editing and the development of cardiovascular disease indicating that a single epitranscriptomic RNA modification can maintain cardiovascular health. Synopsis RNA-editing of Filamin A pre-mRNA is decreased in human cardiac disease. A mouse model lacking this editing site shows altered smooth muscle contraction and diastolic blood pressure, illustrating that ADAR2-dependent RNA editing plays a functional role outside the central nervous system. The Filamin A (FLNA) pre-mRNA is subject to RNA editing with the highest rates seen in the cardiovascular system. FLNA editing rates are reduced in cardiovascular disease patients. In mice, FLNA editing controls smooth muscle contraction of the dorsal aorta. Mice deficient in FLNA editing show elevated diastolic blood pressure and cardiac remodeling. Introduction Adenosine-to-inosine (A-to-I) RNA editing is the most prevalent epitranscriptomic change in mammalian RNAs (Nishikura, 2010). As most cellular machineries including translation interpret inosines as guanosines, A-to-I editing can recode mRNAs to produce the translation of novel proteins, not encoded in the genome (Nishikura, 2010; Pullirsch & Jantsch, 2010). A-to-I editing is catalyzed by adenosine deaminases acting on RNA (ADAR) that recognize double-stranded and structured RNAs (Nishikura, 2010). In mammals, ADAR1 and ADAR2 mediate all editing events. ADAR1 is expressed in all tissues and likely targets repeat-derived double-stranded (ds) RNAs. In contrast, ADAR2 shows its highest expression in the brain and can edit coding and non-coding regions of mRNAs (Riedmann et al, 2008; Nishikura, 2010). Most mammalian recoding edits known today affect mRNAs encoding ion channels and receptors within the central nervous system (Hoopengardner et al, 2003; Savva et al, 2012; Li & Church, 2013). Consequently, ADAR2-mediated recoding events were believed to mainly occur in nervous tissue. Impaired editing in humans is linked to neuronal disorders, type I interferonopathies, and cancer (Paz et al, 2007; Rice et al, 2012; Chen et al, 2013; Paz-Yaacov et al, 2015). One conserved mammalian editing substrate encodes the actin crosslinking protein Filamin A (FLNA; Stossel et al, 2001; Levanon et al, 2005). FLNA is composed of 24 Ig-like domains organized in two rod-regions separated by a hinge (Fig EV1). FLNA homo- and heterodimerizes with the paralogous protein FLNB via its 24th C-terminal Ig-repeat, while the N-terminal region mediates actin binding (Stossel et al, 2001; Robertson, 2005). Loss of FLNA in mice causes vascular abnormalities and reduced vascular tension (Feng et al, 2006; Hart et al, 2006; Retailleau et al, 2016). Exon 42 editing induces a Q-to-R amino acid exchange in Ig-repeat 22 in a region that can interact with over 90 proteins (Stossel et al, 2001; Levanon et al, 2005; Zhou et al, 2010; Nakamura et al, 2011; Fig EV1). In mice, FLNA editing primarily occurs in the vasculature and the digestive tract, which makes FLNA the first prominent recoding event outside the nervous system (Levanon et al, 2005; Stulic & Jantsch, 2013). Click here to expand this figure. Figure EV1. Scheme of Filamin A structure and editing-induced amino acid exchange Filamin A protein is composed of 24 Ig-like repeats. Editing changes Q2341R amino acid in repeat 22 from a glutamine (Q) to an arginine (R), as indicated. Interaction partners to the respective regions are indicated above. The amino terminus harbors an actin-binding domain, while the C-terminal end is interacting with integrins, RhoA, Caveolin, Pak1, Smad2, or ROCK. Repeat 24 is required for homo- and heterodimerization with Filamin B. Download figure Download PowerPoint Here, using large-scale publically available control and patient transcriptome data sets, we show that FLNA editing mediated by ADAR2 in human cardiovascular tissues exceeds the total ADAR2 editing activity previously identified in nervous tissue making it the prime editing target. Importantly, samples derived from cardiovascular patients show a dramatic reduction in FLNA editing in cardiovascular tissues. To explore the function of FLNA editing in the cardiovascular system, we generated transgenic mice impaired in FLNA editing. These mice show increased vascular contraction, elevated blood pressure, arterial remodeling, and left ventricular wall thickening, which eventually leads to left ventricular hypertrophy and cardiac remodeling. Thus, we establish the biomedical impact of a single RNA editing event and reveal a putative biomarker or therapeutic handle. Results FLNA editing is highest in cardiovascular tissue and significantly reduced in patients with cardiac disease mRNA recoding by ADAR2 is typically a brain-specific phenomenon that can diversify receptor function (Holmgren & Rosenthal, 2015). However, the availability of large-scale transcriptome data permits detailed analyses to revisit this long-held assumption. The GTEx database offers high-quality transcriptome data from dozens of tissues from hundreds of donors (GTEx Consortium, 2013), which enables a comprehensive survey for the expression and activity of editing enzymes. Unexpectedly, we found the highest expression levels of ADAR2 in the tibial artery, aorta, coronary arteries, and other vascular tissues, far exceeding the previously reported prominent ADAR2 expression in the nervous system (Fig 1A and Appendix Fig S1; Melcher et al, 1996). We found ADAR1 and ADAR3 expressions match the previously reported ubiquitous and prevalent neuronal expressions, respectively (Kim et al, 1994; Chen et al, 2000; Appendix Fig S1). Figure 1. FLNA editing in cardiac patients from GTEx donors ADAR2 is most strongly expressed in vascular tissues. Graph showing ADAR2 gene expression data derived from GTEx for few representative human tissues. Note the highest expression of ADAR2 in the tibial artery followed by colon and esophagus. Boxes represent the 25th and the 75th percentile with median represented by the black line in the box. The whiskers depict the minimum and the maximum value. FLNA gene expression in few representative human tissues from > 500 different donors using GTEx data. Tibial artery, colon, and esophagus show highest gene expression similar to ADAR2 expression. Boxes represent the 25th and the 75th percentile with median represented by the black line in the box. The whiskers depict the minimum and the maximum value. Bar graph shows FLNA RNA editing (%) among few representative human tissues. Note very high editing levels in the arterial system (red bars). Scatter plot shows the log fold change in editing levels of several candidates between ventricles of healthy donors and heart samples of cardiomyopathy patients. FLNA marked in the graph is one significant discriminator. Y-axis is the −log 10 of the P-value for the difference between healthy and sick. The colors (red vs. green) reflect the threshold cutoffs randomly assigned to distinguish the sites, which demonstrate large, highly significant differences between the two groups. Fold change cutoff of 0.9 was used. Scatter plot shows the FLNA RNA editing in control and diseased human tibial arteries and aortae. At least four control and seven diseased human donors were used in each case. P-value < 0.05 measured by t-test was considered significant. Source data are available online for this figure. Source Data for Figure 1 [embj201694813-sup-0006-SDataFig1.xlsx] Download figure Download PowerPoint We then used the GTEx RNA-Seq data to comprise a list of 252 putative editing sites located in coding sequences to identify the main editing targets in the cardiovascular system (Table EV1). A cluster analysis of editing substrates and levels within the cardiovascular system demonstrates that the appendage and ventricle cluster together, as well as tibial artery, dorsal aorta, and coronaries (Appendix Fig S3). Among these candidates, we found Filamin A was highly edited, up to 98% in the aorta and coronary and tibial arteries (Fig 1C and Appendix Fig S2), in which FLNA has an extremely high expression level (Fig 1B and Appendix Fig S2). When total A-to-I editing levels were further compared for the 38 most highly edited sites between vascular and nervous tissue (cerebellum), much higher editing levels were found in vascular than in neuronal tissue (Appendix Fig S4A). In fact, editing of Gria2, the previously considered most abundant ADAR2 target, only ranks at position 9, almost two orders of magnitude below FLNA (Appendix Fig S4B and Appendix Table S1A and B). This shows that editing in coding regions is most abundant in the vascular system, massively affecting previously less considered substrates such as FLNA or IGFBP7. So the FLNA transcript seems a prime substrate of ADAR2 across all tissues, exceeding the editing reactions at all previously known brain-related sites. Further, FLNA editing and ADAR2 expression show a good correlation in the vasculature (Fig 1, and Appendix Fig S4 and Appendix Table S1, and Table EV1). To assess the putative impact of these abundant editing events in vascular tissues on cardiovascular health, we analyzed the five cardiovascular tissues available in GTEx (aorta, tibial artery, coronaries, left ventricle, and left atrial appendage yielding 1,111 samples derived from 478 donors) for sites of robust A-to-G editing. We divided this cohort into 268 donors with cardiovascular conditions and 210 donors without cardiovascular conditions according to the provided health records. We also scanned NCBI's SRA databank for sequencing data from heart failure patients and recognized a suitable cohort (Schafer et al, 2017). We then compared editing rates between these cardiomyopathy patients and the healthy GTEx cohort and selected editing sites that demonstrated remarkable (> 1.9 fold) differences in editing rates between healthy subjects and patients with dilated cardiomyopathy (DCM; Appendix Table S2). Doing so, the most significant change in editing was found in the RNA encoding SON. Here, editing leads to a synonymous codon exchange, which will not affect the encoded protein. The most significant change in editing leading to a non-synonymous codon exchange occurred at the conserved editing site in Filamin A, which gives rise to a Q2341R amino acid exchange (Fig 1D). Average editing levels in FLNA mRNA dropped by half from 23% in 69 samples to 12% in 112 heart samples of patients suffering from dilated cardiomyopathy (P = 2.237471e-06) (Schafer et al, 2017) in the SRA cohort when compared to the unaffected GTEx samples (Fig 1D). We found that FLNA was also a significant discriminator between healthy individuals and those suffering from a cardiovascular disease within the GTEx cohort itself (23 vs. 17%, respectively, P = 0.003, Table EV2). Filamin A editing levels in the vascular tissue are even higher than in the ventricle. Thus, we compared editing rates from tibial arteries and aortae from fresh cadavers with visible cardiac hypertrophy or aneurisms with those from cadavers with no record or signs of cardiac pathologies (LV hypertrophy, aneurism). Interestingly, a striking difference was observed in tibial arteries where average editing rates dropped from 87.8 ± 3.7% in control samples to 68.5 ± 12.5% in donors showing dilated cardiomyopathy due to cardiovascular disease while editing rates in aortae dropped from 77.5 ± 9.1 to 62.6 ± 11.8% (Fig 1E). However, it should also be noted that editing levels stayed normal in some samples showing cardiac pathologies indicating that not every cardiac pathology is accompanied by a drop in FLNA editing. Next, we tested whether the drop in editing levels could be correlated with ADAR2 expression levels. As shown in the correlation regression plots in Appendix Fig S4C, no significant correlation was found between ADAR2 expression and FLNA editing in tibial artery and aorta from healthy and diseased cadavers. This is in contrast to the GTEx data where a good correlation between the two parameters was seen when different tissues were compared (Fig 1A–C). Taken together, these data demonstrate a correlation between a drop in FLNA editing and cardiovascular pathologies. Creation of mice deficient in FLNA editing We then sought to determine whether changes in Filamin A editing have a causal effect on the development of cardiovascular pathologies. We generated a mouse with an exclusive deficit in FLNA editing. We disrupted the double-stranded structure required for editing by removing the editing complementary site (ECS) in intron 42 from the X-linked Filamin A gene via homologous recombination in ES cells (Fig 2A). The resulting hemizygous males and homozygous females were devoid of FLNA mRNA editing (Fig 2B). These mice designated as FLNAΔECS did show normal FLNA expression levels as judged by qPCR (Fig 2C), Western blot (Fig 2D), and RNA-Seq (Appendix Fig S5A). As FLNA and FLNB can heterodimerize and have similar functions, we also examined the expression and editing of FLNB. However, neither FLNB expression nor FLNB editing was significantly affected in the absence of FLNA editing (Appendix Fig S5B). We observed no apparent abnormalities and normal life expectancy and fertility in both male and female FLNAΔECS mice. Due to the X-linked nature of the FLNA gene, we primarily examined male littermates, which only carry a single allele for further analysis. Figure 2. Generating mice deficient in Filamin A editing Scheme showing the FLNA wild-type (wt) allele, the targeting vector, and the FLNAΔECS neo-allele. The loxP flanked PGK-neo cassette in the targeting vector replaced the editing complementary sequence (ECS) using homologous recombination, which was then deleted using Cre recombinase. Right, Southern blotting analysis screened for positive clones shown. The positions of loxP sites, restriction enzyme (EcoRI), and Southern blotting probe are also indicated. Sequencing electropherograms show the average FLNA editing levels in wt and FLNAΔECS tissues (stomach, colon, heart, lung, and tibial artery). Editing levels were checked in tissues from three independent mice, and the value below the chromatogram depicts the average value of three replicates. FLNA mRNA expression levels measured by qPCR in wt and FLNAΔECS colon tissue showed no difference. FLNA protein levels measured by Western blotting are identical in wt and FLNAΔECS stomach tissue. Y-axis represents FLNA expression normalized to tubulin levels. FLNA editing levels (%) in wt whole dorsal aorta, tunica media, and tunica adventitia. Tunica media consisting of smooth muscle cells show the highest FLNA RNA editing. Data information: Arrows indicate the FLNA editing site. For (C–E), data are shown as mean ± SD from three independent experiments. **P < 0.05. Source data are available online for this figure. Source Data for Figure 2 [embj201694813-sup-0007-SDataFig2.zip] Download figure Download PowerPoint Absence of FLNA editing increases smooth muscle contraction In mice and humans, FLNA editing is highest in vascular tissues and other organs rich in smooth musculature (Stulic & Jantsch, 2013; Fig 2). Separation of mouse dorsal aortae verified that the smooth muscle layer (tunica media) displays significant FLNA editing levels (> 90%) compared to the 60% editing levels in the whole aorta (Fig 2E). Since FLNA crosslinks actin and interacts with regulators of smooth muscle cell contraction, such as RhoA and ROCK (Nakamura et al, 2011), we tested whether FLNA editing affects smooth muscle contraction. We tested rings of dorsal aortae in myograph chambers. Indeed, FLNAΔECS aortic rings showed a significant increase in smooth muscle contraction upon treatment with the thromboxane A2 receptor agonist U46619 compared to wild-type (wt) mice (Fig 3A). Even though Emax increased, the EC50 remained unaltered (Fig 3A). Both maximum contraction and contractile force increased by 30% in aortae of FLNAΔECS mice (Fig 3B). After U46619 administration, primary vascular smooth muscle cells (vSMCs) from FLNAΔECS mice also hypercontracted compared to wt vSMCs with a comparable dose–response (Fig 3C and D). Together, these results indicate that FLNA editing regulates the contraction of smooth muscle cells. Figure 3. FLNAΔECS aortae and primary vSMCs show hypercontraction in response to U46619 A, B. Treatment of aortic rings with thromboxane A2 receptor agonist U46619 leads to (A) hypercontraction and (B) increased contraction force in FLNAΔECS aortae in myograph chambers. Emax was higher in FLNAΔECS aortae without any difference in EC50 as compared to wt aortae. For each condition, 10–12 aortic rings from at least four wild-type (wt) and four FLNAΔECS mice were used. Data are shown as mean ± SEM. *P < 0.05, **P < 0.01, ***P < 0.001 (Student's t-test) C. Graph shows the normalized cell contraction measured by xCELLigence Real-Time Cell Analyzer in wt (red) and FLNAΔECS (green) primary vascular smooth muscle cells (vSMCs) indicating hypercontraction i
更多查看译文
关键词
<scp>mrna</scp>,<scp>rna</scp>,blood pressure,vascular contraction
AI 理解论文
溯源树
样例
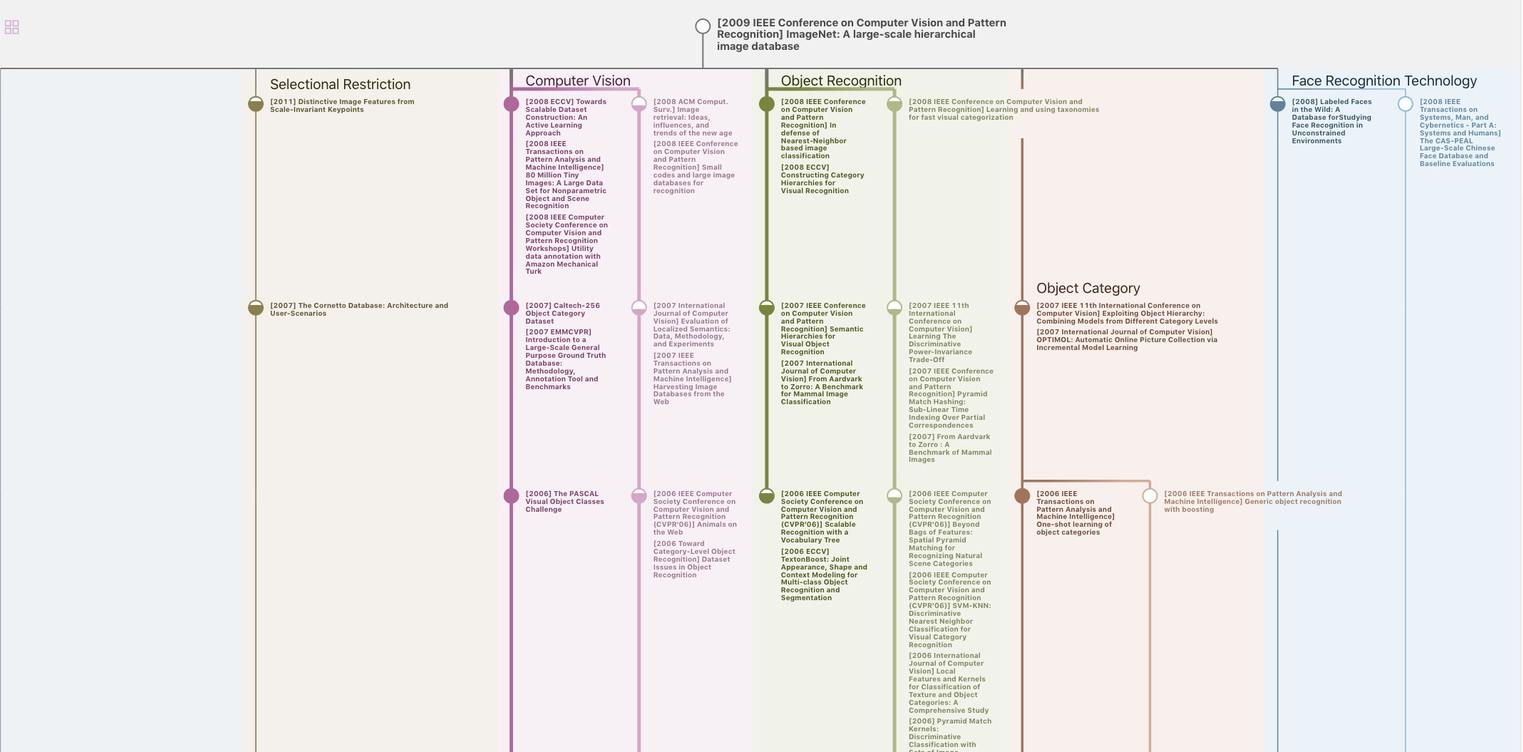
生成溯源树,研究论文发展脉络
Chat Paper
正在生成论文摘要