Alternative Strategies for Emergency Blood Transfusion in Low‐resource Settings: A Scoping Review
Transfusion(2024)
摘要
Hemorrhage and anemia represent a common cause of preventable death.1 Although the exact number of deaths due to hemorrhage is unknown, at least 5 million people worldwide die every year from trauma-related injuries, with roughly 40% of those deaths estimated to be secondary to bleeding or its sequelae.1, 2 Bleeding accounts for 25% of maternal fatalities in Africa and3 50-80% of pediatric sickle cell anemia patients in Africa die before the age of five, often without access to adequate blood.4 While blood is an essential therapeutic, the logistics of blood banking required to deliver safe and timely blood are complex and expensive. Unsurprisingly, the global blood shortage disproportionately affects low- and middle-income countries (LMICs) with most of sub-Saharan Africa (SSA), Oceania, and South Asia.5 The challenges are multifactorial and well documented, ranging from low blood donation rates, a high prevalences of anemia and transfusion transmissible infections, limited testing availability, and insufficient financing of the blood banking infrastructure.6, 7 While the global health community and transfusion experts across the world have been working for decades to narrow the gap between the demand and supply of blood, progress continues to be incremental. Large parts of the world, particularly rural areas, continue without access to any blood banking infrastructure. These regions have been referred to as "blood deserts."8 To compound challenges, the U.S. government's President's Emergency Plan for AIDS Relief (PEPFAR), a major funding body for transfusion services in many LMICs, is curtailing some funding streams.9, 10 To meet blood needs, innovative strategies that do not rely on the traditional process of blood collection, processing, and delivery are urgently needed.11 Some proposals have included walking blood banks (WBBs), autologous transfusions, hemoglobin oxygen carriers, drones for blood delivery, and mobile phone-based recruitment of donors for emergency blood transfusion, but little is known as to their application in low-resource settings in LMICs.12 We conducted a scoping review of the literature on innovative, alternative strategies for emergency blood transfusion in low-resource or austere settings to understand the feasibility or efficacy of strategies that are in development for application in a low-resource setting or have already been studied in such settings. This scoping review was carried out in line with PRISMA-ScR guidelines (Supporting Information checklist).13 Before performing the literature search, the steps of the study were planned and written in a protocol as outlined below. No ethics or Institutional Review Board approval was sought for this study as it involves neither human participants nor unpublished primary or secondary data, and thus, it is not applicable. There was no patient or public involvement in this study as this is a scoping review study. We reviewed published literature across five databases in December 2020, including PubMed, Scopus, MEDLINE, EBSCO Host: Global Health, and Web of Science (Table 1). Among the search terms used were "blood transfusion," "blood substitute," "transfusion alternative," "freeze dried plasma," "hemoglobin based oxygen," "unmanned aerial vehicle*," "unbanked." This was combined with a standard set of terms around wording for LMICs and LMIC Countries. All resulting searches were uploaded into Covidence software (Veritas Health Innovation Ltd, Melbourne) for removal of duplicates, title and abstract screening, and full-text review, and data extraction. The full search strategy for PubMed is included in Appendix S1. For articles not available to download, multiple efforts were made to obtain them through ResearchGate, the Harvard library system, and contacting authors by email. We included all studies that referred to a method of blood transfusion in an LMIC or austere setting or military settings, for urgent medical or surgical conditions and that did not describe a typical blood banking process. We included all article types, including primary research and editorials, news articles, opinion pieces, reviews, and policy papers. This was to better assess the full scope of literature available on these interventions. We excluded the use of drugs, hemostatic agents, campaigns for increasing blood donations or banked blood, research on the prevention of hemorrhage, blood management systems, and other methods utilizing optimization algorithms for blood use. Further, we excluded all studies conducted in HIC settings, those regarding non-emergent or chronic conditions, animal studies, and articles not in English or Spanish. For title-abstract and full-text screening, two reviewers screened each article independently, and a third reviewer resolved conflicts. Two reviewers extracted data from all studies separately which then went through a consensus process using Covidence's consensus tool.14 We extracted standard article data on the country of the first and last author based on institution, publication date, and study type. In regards to the alternative technology, we extracted data on the type of technology, the country in which it was studied, the patient population studied, the number of patients included, inclusion and/or exclusion criteria if any, whether the study reported patient outcome data, cost of the alternative technology, and if applicable, in-hospital mortality, 30-day mortality, and post-transfusion reactions or infection on follow up. Five articles mentioned two technologies, and each technology was analyzed as a separate entry. The extracted data was exported to an Excel file for analysis. We compiled descriptive analyses of alternative technologies, types of studies, study settings, patient outcomes, and the cost of each technology. Our search generated 14,379 studies, 7320 of which were duplicates. We reviewed 7056 titles and abstracts, and then 207 full texts, 92 of which were included in the data extraction phase. We were unable to find the full texts for 15 studies. The full PRISMA flow diagram can be seen in Figure 1. A breakdown of the search and results from each database can be seen in Table 1. Thirty-five articles reported primary patient outcome data through retrospective review (N = 13), prospective observational study (N = 6), non-randomized experimental studies (N = 2), randomized control trials (N = 2), or case reports (N = 10), or series (N = 2). Thirty-six articles were review articles with only three of those as systematic reviews. There were three qualitative studies, two cross-sectional studies, and one book chapter. There were 10 opinion pieces in total, including editorials (N = 5), expert opinions (N = 3), and a letter to the editor (N = 1). Finally, there was also one quality improvement initiative, one novel device description, and four news articles. Thirty-seven articles reviewed or reported on military data, which primarily reported on male patients with combat injuries aged 20–35 years old. We did not cross-reference the review articles' citations for overlap with the primary articles included in the review section. Our search generated a total of seven technologies that were included in the data extraction and analysis. Table 2 outlines the technologies included and their respective breakdown of article type, geographic setting, population(s) studied, and first/last author country. Figures 2 and 3 break down into heat maps, the location of where studies occurred and the locations of first authors. The full table of all articles included in this scoping review is included in Appendix S2. 14 Review article 6 Case report 1 Case series 5 Cohort study (retrospective) 2 Cohort study (prospective) 2 Editorial 1 Cross sectional study 1 News article 1 Retrospective review + review article 1 Quality improvement initiative 1 Letter to the editor 1 Qualitative research 1 Device description 8 Afghanistan 6 Iraq, Afghanistan 6 Iraq 5 India 1 Bangladesh 1 Iraq, Afghanistan, Vietnam, Korea 1 Haiti 1 Nigeria 1 Kosovo 1 At Sea 1 Syria 5 N/A 21 Military 4 mixed military & civilian 1 newborn 1 obstetric care providers 1 civilian adult 9 N/A or unknown 19 USA 3 UK 3 USA/India 2 India 1 France 1 Bangladesh 1 Canada 1 Poland 1 Norway 1 Germany 1 Israel 1 Nigeria 1 South Africa 1 Luxembourg 8 Review article 3 Cohort study (prospective) 5 Cohort study (retrospective) 2 Systematic review 2 Randomized controlled trial 2 Qualitative research 1 Device description 1 Non-randomized experimental study 2 Case report 4 India 3 Nigeria 2 China 2 Sub-Saharan Africa 1 Afghanistan 1 USA 1 South Africa 1 Ghana 1 Haiti 1 Zambia 1 Tanzania 1 Uganda 1 Ghana, India, Iran, Kenya, South Africa, Turkey, Uganda 6 N/A 6 Obstetric patients 4 Adults 4 All patients 2 Patients undergoing cardiac surgery 1 Combat injury 1 Trauma 1 Surgery and anesthesia providers 1 Patients with RhD-negative blood 6 N/A 5 Nigeria 5 India 3 USA 2 South Africa 1 China 1 Uganda/Netherlands 1 China/USA 1 Ghana 1 Israel 1 Zambia 1 Tanzania 1 Uganda 1 Sweden 1 Netherlands 1 UK 7 Review article 3 Editorial or Commentary 3 Expert Opinion 2 News article 1 Book chapter 1 Systematic review 4 South Africa 13 N/A 2 Adults 1 Military 14 N/A 11 USA 2 South Africa 1 South Africa/USA 2 Canada 1 Germany 5 Review article 3 Case report 1 Cohort study (prospective) 1 Cohort study (retrospective) 1 Editorial 3 Afghanistan 2 Israel 1 Morocco 1 Djibouti 4 N/A 5 Military 3 Pediatrics 1 Combat injuries—mixed military and civilian 2 N/A 5 USA 2 Israel 1 UK 1 Sweden 1 France 1 Djibouti 1 Cohort study (retrospective) 1 Review article 1 Case series 1 Cross sectional study 2 Afghanistan 1 India 1 United States 2 Adult trauma 1 Military 1 Adult and pediatric civilians 1 Netherlands 1 India 1 Australia 1 USA 1 News article 1 Review 1 Rwanda 1 N/A 1 India 1 Canada A WBB refers to the process of just-in-time emergency transfusion where blood donors are summoned, on-demand from often a pre-defined donor pool; the collected blood is tested with an expedited testing process that often consists of rapid diagnostic testing (RDT) for donor qualification and donor-patient blood compatibility. Therefore, fresh whole blood is transfused directly instead of first separating it into components. Variations of this WBB process have been used for decades either officially or unofficially and have also taken the name of "unbanked direct blood transfusion" or "emergency donor panels." WBBs attempt rapid mobilization of blood units for on-demand availability in rural and remote settings in areas without access to a fully functioning blood bank with stored blood.15 Most iterations of WBBs rely on fresh whole blood (FWB) in the context of life-threatening hemorrhage. WBBs have been used by militaries across the world, including the United States. The U.S. military has given thousands of safe and effective fresh whole blood transfusions in this context in conflicts in Iraq and Afghanistan, described further below.16 The risk of undetected transfusion-transmitted infections (TTIs) is most often cited as the major drawback, as RDTs are described as having lower sensitivity and specificity for HIV, hepatitis B, hepatitis C, and syphilis than enzyme-linked immunosorbent assay (ELISA), which is often the standard in most LMIC blood banks.17, 18 However, studies are lacking on the sensitivity of RDTs compared to the standard immunoassays used in LMICs.17, 18 Kannangai et al. and Lien et al. have demonstrated that there is no greater risk of morbidity or mortality with fresh versus banked blood.17, 18 The RDTs used for WBBs have been used in South Asia and Africa by hospital blood banks due to resource limitations. This practice is recognized and acknowledged as necessary by the WHO and various national transfusion services.19 The risk of increasing transfusion infection transmission through the blood supply would be small compared to the risk of morbidity or mortality from blood unavailability when needed for transfusion.18, 20-25 A study of American military personnel who received battlefield transfusions revealed only one transfusion-transmitted hepatitis C infection, which corresponds to an incidence rate of 2.1 per 1000 persons.21 Studies in combat military settings in Afghanistan demonstrated a low rate of hemolytic reactions from ABO incompatibilities.19, 20 While the studies address aspects of WBB's performance in the military context, some articles addressed its use in the LMIC civilian context. In a letter to the editor, a clinician from South Africa discusses family-directed blood donation in Malawi, Papua New Guinea, and South Africa, where social ties are leveraged for blood donation and can be important for rare blood types.24 The Smart Blood Query project builds on this concept and uses Rapid SMS, an open-source short message service (SMS) in Southeast Asia to connect a database of pre-screened donors to increase blood donation during disasters and otherwise, allowing both donors and recipients to access the platform in times of crisis, making it amenable to just-in-time processes like WBB.25 Autologous blood transfusion refers to the collection of blood from a patient and its re-transfusion into the same individual at a later time.26 Three main techniques for autologous transfusion are—predeposit autologous donation (PAD), acute normovolaemic hemodilution (ANH), and perioperative cell salvage (PCS).27 PAD involves drawing blood from the patient weeks before planned surgery, which is stored until use.28 In ANH, the blood is collected in the operating room after the patient is under anesthesia but before the operation begins in standard bags containing anticoagulants on a tilt rocker with automatic volume sensors. The removed blood volume is replaced with a plasma substitute. ANH aims to lower circulating blood cells by dilution, which leads to decreased blood loss in the case of intraoperative blood loss, which can then be replaced with full-concentration blood collected from the patient immediately prior.26 There is limited information on the cost-effectiveness of the ANH machines or their applicability to low-resource settings. In PCS, blood that is lost during the operation is collected, filtered, and transfused back to the patient within 6 h, either intraoperatively or immediately post-operatively.26, 29 The most commonly cited indication for autologous transfusion in our results was anticipated significant blood loss during surgery under semi-controlled circumstances, for example, during a major cardiac procedure or orthopedic operations. PCS was particularly useful in traumatic injuries that resulted in significant exsanguination or anemia, or in situations where blood is not immediately available (e.g., remote rural areas), cases with clinical contraindication to blood transfusion (e.g., previous history of severe transfusion reaction) or where the patient is unwilling to receive allogenic blood products (e.g., Jehovah's Witness religion). Sjoholm et al. interviewed surgery and anesthesia providers in low-resource settings and highlighted their opinion that autologous transfusion is under-utilized in resource-limited settings with significant opportunities for growth in the setting of penetrating trauma and obstetric emergencies.29 Benefits of autologous transfusion include decreased risk of transfusion-transmitted diseases, red cell alloimmunization, and transfusion reactions related to allogenic blood transfusion. Solomon et al. in South Africa showed that intraoperative cell salvaging is feasible and effective and even saved the equivalent of 186 units of RBCs in 80 patients over 1 year29, 30 In our review, we found no studies reporting in-hospital mortality or significant transfusion-related reactions in association with autologous transfusion. However, the studies reported a few notable concerns with cell salvaging. First is the risk of contamination of salvaged blood by enteric organisms, although one study has demonstrated no difference in septic complications.31 Second, autologous transfusion efforts require a focused strategy for its implementation, including processes, equipment, consumables, and trained personnel.30 When the costs of practicing intraoperative CS at two South African hospitals were compared to the costs of the equivalent amount of blood purchased from the National Blood Service, CS was marginally more expensive.30 Hemoglobin-based oxygen carriers (HBOCs) are made of modified human, animal, or recombinant hemoglobin that act as a substitute for transporting oxygen to tissues of the body. This can reduce oxygen deficiency and attempts to avoid or reduce the need for blood transfusion. They are available in stable formulations with long shelf life and do not require cross-matching.30 Plasma residence times for HBOCs range from approximately 12 h for cross-linked hemoglobin to about 2 days for polyethylene glycol (PEG)-hemoglobin. In contrast, human red blood cells have a mean residence time of 120 days.31, 32 Even though their plasma residence times are significantly shorter than RBCs, they can be considered as a stop-gap measure of oxygen delivery until appropriate units of RBCs are found. Side effects of HBOCs include vasoconstriction, hypertension, gastrointestinal complaints, and jaundice with mild elevation of liver enzymes.32-34 Levien et al. also found a greater risk of myocardial infarction in patients treated with HBOCs.35 Moreover, the cost of these products can be a serious impediment to their widespread use. Due to identified significant physiological risks and limitations, HBOCs are mainly reserved for specific cases such as preventing death from anemia or hemorrhage in individuals adhering to the Jehovah's Witness religion when no other alternatives exist.34, 36 There is limited information available about the cost of these products or their applicability for low-resource settings.31, 32, 34, 36 Freeze-dried plasma (FDP) is prepared by lyophilizing, also known as freeze-drying the plasma after separating it from fresh blood. Its advantages over thawed FFP include its stability at ambient temperatures and long shelf life, universal blood group compatibility due to antibody dilution and neutralization, and ease of transportation and logistics of administration.37 The utilization of FDP allows for the rapid provision of beneficial plasma, while FFP requires initial thawing, a process that can take up to 30 min and potentially delay resuscitation.37 In less than 6 min, FDP can provide up to 210 mL of a ready-to-use hemostatic that can provide fibrinogen and clotting factors at levels that are equivalent to fresh frozen plasma (FFP).37, 38 A noteworthy decrease in PT (p < 0.01) was observed, with an average reduction of 3.3 s. Users of FDP reported a straightforward application, clinically observed effectiveness on par with FFP, and the absence of any adverse effects linked to the use of FDP. There were no adverse effects reported in the studies by Martinaud, Rottenstreich, and Nadler attributed to the plasma product. A prospective study on the military population in Afghanistan reported that it was easy to use, was clinically equivalent in efficacy to FFP, and did not report any adverse events attributed to it.37 One study was a systematic review from 2019 from Israel of pediatric trauma patients treated with FDP in the prehospital environment.39 Five out of eleven studies on FDP reported its usage within low-resource settings. Of these, three were from low-income countries while two were from low-middle-income countries (Table 2). FDP demonstrates clinical efficacy comparable to FFP, offering advantages of extended shelf life and straightforward reconstitution logistics. While promising for emergency use in remote areas without blood banks and reliable refrigeration, there is a lack of studies applying this technology in low-resource contexts, necessitating further research, particularly in assessing cost and product performance in such environments. Frozen blood products are red blood cells, plasma, or platelets are cryo-preserved, thawed, washed free of cryoprecipitates, and transfused with a survival of 85%–90% of cells.40 The articles summarize that deep-frozen red cells can be stored at −80°C for at least 10 years, deep-frozen plasma (DFP) for 7 years, and deep-frozen platelets for 2 years. DFP is stored at −80°C and its performance after a 30-min thaw is akin to fresh frozen plasma. Leukocyte-depleted, volume-reduced frozen platelets are stored at −80°C.41 Frozen blood products have had a low incidence of transfusion reactions, and similar mortality and blood product usage to that of trauma patients treated with non-frozen blood products. Additionally, the products are ABO-compatible and tested for transmittable infections. Furthermore, long-distance travel can occur without the need for electricity, as products can be transported on dry ice, and there can be better management of available products with longer expiration dates.40 The current major concerns for frozen blood products were the highly technical methods of glycerolization or deglycerolization, and the equipment necessary for the process, limited 24-h post-deglycerolization shelf life of RBC, need for large amounts of crystalloid solution, long thaw time (up to 1 h), varying efficiency levels of RBC recovery, and a high cost of production.42, 43 However, newer methods of glycerolization and deglycerolization have helped lengthen the shelf life to 14 days and reduce cost.43 Three out of four studies on Frozen products reported its usage in low-and middle-income countries (India and Afganisthan) (Table 2). While the concept of employing frozen blood products to alleviate blood shortages in low-resource areas is appealing due to extended shelf life, the practical challenges associated with logistical complexities in thawing and administration, along with the necessity for sophisticated equipment in processes like glycerolization, impede their viability in emergencies within these settings. An unmanned aerial vehicle (UAV) is defined as a "powered," aerial vehicle that does not carry a human operator, uses aerodynamic forces to provide vehicle lift, can fly autonomously or be piloted remotely, can be expendable or recoverable, and can carry a lethal or nonlethal payload. With recent advancements, unmanned aerial vehicles (UAVs or drones) have been used to overcome geographical barriers, reduce carbon emissions, reduce emergency response complexity, and may also improve the cost-effectiveness of service delivery. Drones can travel long distances relatively quickly and reach geographically isolated areas, bypass obstructive road conditions and closures that compromise standard delivery networks, and remove the human risk of transport in unsafe scenarios be it extreme weather or conflict zones. In our search, we found two articles that spoke to the use of drones to transport blood. One was a news article reporting on the new use of drones to transport blood products in Rwanda, allowing physicians to treat patients in need of blood transfusions at regional centers, instead of needing to be transported to Kigali, the capital. It highlighted that not needing a cold chain to store the blood products, as they were available on demand, allows for the treatment of conditions such as post-partum hemorrhage and anemia.44 The second article, a SWOT (Strengths, Weaknesses, Opportunities, and Threats) analysis of drones, concurred with many of the positive benefits in the news article but also pointed out the concerns around the technical setup needed, the limited carrying capacity of a drone and its safety, community safety, and legal permissions needed from aviation authorities.45 Using UAVs for blood product delivery extends blood banking infrastructure to remote areas, though it requires investments. Enhanced delivery may decrease the necessity for additional blood banks and related investments in resources, potentially supporting other technologies reliant on advanced blood bank processing. Further research on the economics and logistics of implementation and implications for the blood supply is required in more LMICs. In umbilical cord blood (UCB), the blood left over in the placenta and umbilical cord after the birth of the neonate is preserved, and can be used for transfusion in children, particularly infants. UCB is a rich mix of fetal and adult hemoglobin and has low immunogenicity, which reduces the number of immunological reactions. UCB transfusion is a strategy that can be used to address severe anemia in children in areas of blood scarcity. One non-randomized experimental study set in Kenya studied UCB transfusion for children under 12 years old with severe anemia and found a significant rise in hemoglobin of 61 g/L at 28 days after transfusion in children older than 3 months. Even though adverse events were reported, an independent expert panel determined that no serious adverse events or adverse events were caused by cord blood transfusion. Citing these strengths, the study suggested that UCB is efficacious for blood transfusion in infants with a safe side effect profile and has no adverse events attributable to UCB transfusion. However, the study did not report any information on its associated costs or specify the amount of transfused UCB.46 Addressing challenges associated with systematically collecting cord blood units in the context of birthing clinical settings on a broad scale is crucial, as this process is often perceived as problematic. More research is needed as to whether systematic preservation of cord blood in low-resource settings is feasible and could be used to address anemia in infants. The absence of reliable, functional blood banking infrastructure in the world's blood deserts represents a grave threat to health equity and serves as a potent barrier to the provision of high-quality medical care across a range of clinical disciplines from hematology to surgery to pediatrics and oncology. Blood deserts are often found in the world's poorest regions, compounding existing inequities and presenting new challenges for health delivery.5, 8 Urgent efforts are needed to address the crisis with strategies and technologies particularly suited to these contexts. WBBs, autologous transfusion, freeze-dried plasma, and drones are documented among the strategies identified by clinicians, researchers, and innovators to potentially address extreme blood shortages in low-resource settings. Implementation of strategies like frozen blood, UCB, and HBOCs in low-resource settings are currently less promising as HBOCs face financial and FDA approval obstacles.47 Clinical trials for Biopure were halted due to product morbidity, further emphasizing the existing risk–benefit disparity and highlighting the imperative for additional research and development to improve the safety and effectiveness of such strategies.47 By in large, however, the state of research of these technologies for implementation is nascent, with most in the concept stage or demonstrated efficacy in the high-income setting but without rigorous application and evaluation in low-resource settings.8 In our scoping review with a specific focus on low-resource setting applicability, 57 out of 92 studies were not primary research, and the clinical studies that were included were often case reports or series or observational studies. Additionally, heterogeneity in study design and primary outcomes does not allow for a structured comparison between technologies. There is also a paucity of implementation trials with standardized patient-level outcome measures such as intra-hospital or 30-day mortality, analysis of adverse events or transfusion reactions, post-transfusion infection rates, or readmission rates. Given the magnitude of the crisis in global blood deserts, there is a pressing need for active exploration of these novel strategies in high-quality, well-designed clinical trials. Of the strategies identified, the WBB, autologous blood transfusion, freeze-dried plasma, and drone-based blood delivery had the most existing or potential for use in low-resource blood deserts and should be actively explored by clinicians, researchers, policymakers, and funders as potential strategies to address the crisis. WBBs, for example, have been rigorously studied in military settings. WBBs appear to be highly relevant for serious emergencies in the low-resource context when banked blood is unavailable as it requires relatively minimal resources, can be performed quickly, and relies on a whole blood transfusion. While previous attempts at WBB implementation in low-resource settings were hindered by concerns about the sensitivity and specificity of the point-of-care RDTs at the center of its blood screening process, RDTs have improved with time. However, we did not identify any studies that evaluate the safety of this strategy while utilizing the latest generation of RDTs.17, 18 Additionally, the presence of platforms like the Smart Blood Query Project and Damu-Sasa focused on rapid donor mobilization in LMICs is encouraging.25, 48 Implementation science-based demonstration projects with rigorous mixed methods evaluation to assess the utility, feasibility, and safety of this strategy in the low-resource civilian context is urgently needed. Similarly, autologous transfusion particularly perioperative cell salvage- is another strategy established in high-income and low-resource settings that needs additional research for structured adoption in the low-resource context. The benefits of collecting lost blood from the patient and transfusing it back to the patient in the setting of a shortage or absence of banked blood are obvious. No clinical study has examined this process in a low-resource context. Urgent implementation research is crucial, particularly with the advent of newer devices specifically designed for use in such contexts.49, 50 As outlined above, there is a major gap in clinical research for the strategies and technologies identified in this review in study populations and geographic settings relevant to LMICs. The majority of articles focused on populations residing in HICs.12 Annually, just half of the global collected blood goes to just 80% of the global population living in LMICs. Yet, our review found that only 15% of the authors in the articles were affiliated with LMIC institutions.51 Additionally, there were very few studies that mentioned the economic cost or operational implementation of these technologies in LMICs. Many technologies may be suitable for low-resource settings but require a large initial investment related to infrastructure or training. Nonetheless, the benefits in lives saved or even opportunity costs saved from pursuing a traditional blood banking model may easily justify these investments in specific contexts. Nuanced studies understanding the actual needs across these diverse settings and the suitability of particular strategies are needed. The transfusion community should advocate for this research to be done by or in closer partnership with LMIC researchers, who understand the needs of their communities and can devise contextually relevant solutions. Further, 40% of the studies included in this review encompassed the military population predominantly involving young male patients with a traumatic injury. While we may derive many ideas from the military context, which has been at the forefront of research resuscitation strategies for severe hemorrhage, there are several important differences between military and civilian settings, and the "austere" military environment is not always surrogate for LMIC environments, nor can clinical conclusions be generalized across LMIC civilian populations. We did not include articles outside of the English and Spanish languages, and there may be more relevant research out there published in other languages. There were 15 articles (16.3%) for which we could not find the full text despite the aid of librarians and efforts to contact the authors by email or ResearchGate. Although we searched five major databases, other databases and the gray literature could hold additional eligible research. We acknowledge that much of the innovation that occurs in LMIC settings does not get published in peer-reviewed journals due to a spectrum of factors related to research and clinical capacity. For example, there are known technologies related to autotransfusion and donor management systems that have yet to make inroads into the academic literature and hence were not formally included in the review.48-50, 52, 53 We also did not specifically cross-check the citations of the review articles for overlap with the primary articles included in the study results, which could have caused some overlap in information. This scoping review identifies multiple studies with potentially relevant strategies for emergency blood transfusion in the setting of extreme blood shortages and limited blood banking infrastructure in the world's lowest-resource settings. WBBs, freeze-dried plasma, and autologous transfusion have been best identified in this review to aid in overcoming the blood shortage in austere settings. There is a paucity of rigorous clinical, economic, and implementation research on using these strategies in these environments. High-quality, context-specific, holistic research on the feasibility, safety, and implementation of these strategies in low-resource settings is urgently needed. The author declares no conflicts of interest. All data relevant to the study are included in the article or uploaded as online Supporting Information. Appendix S1. Full search strategy for PubMed. Appendix S2. Summary of articles reviewed. Please note: The publisher is not responsible for the content or functionality of any supporting information supplied by the authors. Any queries (other than missing content) should be directed to the corresponding author for the article.
更多查看译文
AI 理解论文
溯源树
样例
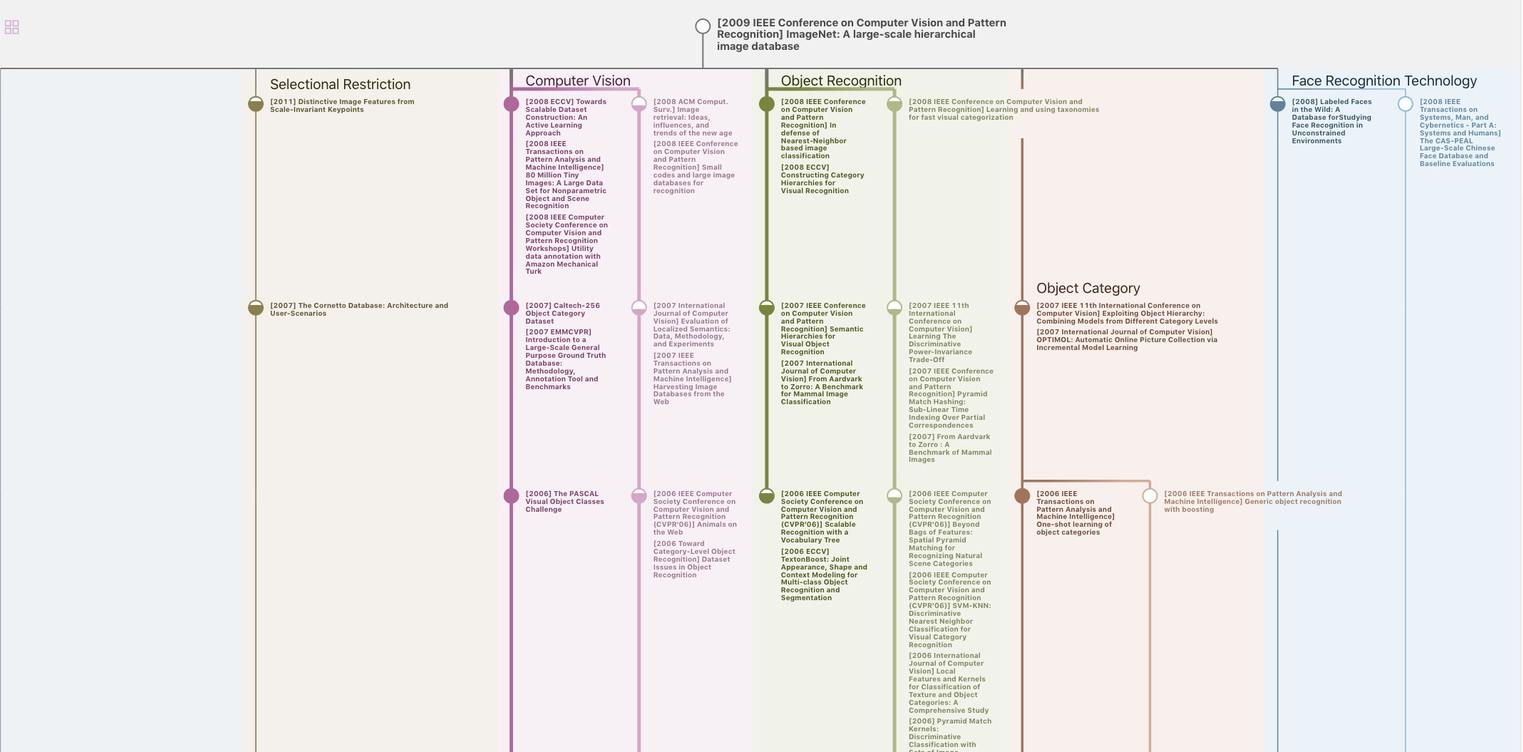
生成溯源树,研究论文发展脉络
Chat Paper
正在生成论文摘要